
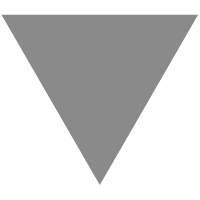
USB Connector Resistance: Another reason for slow phone/tablet charging | Gough&...
source link: http://goughlui.com/2017/11/20/usb-connector-resistance-another-reason-for-slow-phone-tablet-charging/
Go to the source link to view the article. You can view the picture content, updated content and better typesetting reading experience. If the link is broken, please click the button below to view the snapshot at that time.
USB Connector Resistance: Another reason for slow phone/tablet charging
It was back in 2014 when I first published about USB cable resistance as one reason why your phone or tablet might be charging slowly. At the time, quality cables with good gauge wires were hard to find, and many “cheap” 28AWG cables were bought by unsuspecting consumers without knowing the downsides. Since then, it has become one of the most-viewed articles on this site, and the market has responded positively by advertising cable specifications more frequently.
However, this is only one part of the puzzle, and it is something I had realized almost right after I posted that article. Unfortunately, I couldn’t find the motivation to explain the technicalities at the time. Since then, a number of comments and reviews later, I feel that I’m better prepared to explain another issue which has existed since the dawn of USB but has gotten worse as we attempt to push more current through our cables.
Is that USB connector designed correctly?
USB at its introduction specified the ability to transfer up to 500mA at 5V over the USB A and B connectors. Throughout the iterations, the number of connector types have increased, and so has the current demanded by end-devices. USB “legacy” connectors still remain with us today and still remain relevant to many chargers which feature a USB-A socket.
Unfortunately, the design of USB focused on making things cost-effective, and as a result, these legacy connectors tend to be less-accurately specified from a dimensional standpoint. This means that connector sizes can vary significantly between manufacturers, but “it should still work”. How well this works in practice is a different story.
I’m sure that many readers may have experienced a headphone socket which crackles and might need you to turn the plug a little or pull it out slightly before a good connection is made.
Others may have even experienced a USB charging cable which refused to charge until a little lateral pressure is applied to the connector, and then it worked. Or maybe, you needed to pull it out just a tiny bit.
These are all manifestations of contact resistance which may arise due to connector incompatibility.
The design of the USB connector is such that this problem should be minimised in properly-designed connectors.
I will focus on the USB-A connector, although the principles hold true for most types of connectors. The first feature to look at is the shape of each of the pins in the connector. Properly formed connectors have a tapered design at the front, which leads to a “raised ridge” in the centre. This whole pin is gold-plated to avoid oxidation and maintain good contact.
When mated with its appropriate mate which contains gold-plated contact springs, the raised ridges concentrate the pressure to “break through” any surface dirt. The plastic tongues on the undersides provide a stable “backing” and increase the pressure between the spring surface and the contact while enforcing connector alignment. The outer shell keeps the connectors from pulling apart, provides a continuous shielding path and maintains the alignment of the pins.
This all sounds pretty simple, but in reality, I’ve seen a lot of things go wrong:
- Some connectors I’ve seen have absolutely no gold plating whatsoever, which makes them liable to oxidation and potentially depositing dirt onto perfectly-good connectors. Others have false “gold-coloured” plating which wears off after only very few uses.
- Others have tongues which are too “thin” in the vertical dimension, and thus the plug doesn’t make good contact unless it’s tilted downward or upward.
- A cost-saving measure seems to be the use of “thinner” and more “flexible” shell materials for the plug, which are liable to bending and mis-shaping after the application of a mild amount of mechanical stress. This jeopardizes the tightness of the connection and can lead to premature contact wear due to intermittent contact.
- Some are “cost-reduced” in manufacturing resulting in “flat” pin profiles that do not achieve as reliable contact as the raised-ridge profile which it should have. This is common on “PCB” style plugs.
- There are some completely unconventional plugs (e.g. a “reversible A” or a “shell-less A”) which violate the standard and thus rely on other parts of the socket to compensate in order to make the connection work.
Another point is that connectors are not designed to be used forever. Most connectors have data-sheet lifetime specifications of about 10,000 cycles to a maximum contact resistance of 30mohms. This sounds plenty, right? For 10 cycles a day, it’s almost three years to failure.
The truth is that this data-sheet stated lifetime is for ideal circumstances. This means not exceeding any current ratings, using their appropriate mating partner connector, under perfectly clean circumstances with no added mechanical stress. Once you add reality into the mix, these ratings can go right out the door.
For starters, any trapped particles can act as an abrasive compound wearing away the precious gold plating and serve to impact resistance. Any contamination from atmospheric deposition can result in corrosion where the plating is broken. Arcing from “loose” connections due to poor “partner” sockets can destroy the coating. Damaged sections increase contact resistance. Once you add the possibility of the outer shell being dimensionally affected by bending due to stress, a good connection is hard to ensure.
Hopefully, now, you can begin to appreciate that a USB connector is not as simple as it appears. But you might not be convinced that this is a problem.
A look at the cable resistance shows that for a 1m 24AWG cable, the cable contributes about 168mOhm of resistance. The connectors are supposed to be up to 30mOhm each at the end of life, or 60mOhm total. This isn’t entirely negligible, especially if we improve the cable. A better cable can be hamstrung by poor connectors!
“QC2.0/3.0 and higher voltages eliminates the problem!” Really?
One of the biggest push-backs I’ve had in regards to the original cable resistance article is a misconception that higher charging voltages solves the problem. The problem is that there are two problems in reality and neither of them are completely solved by higher voltages.
The first problem is under “regular” 5V charging, some devices may show slow charging or no charging at all when connected to long cables. When they upgrade to a QC2.0 charger, they see the device can now take a charge, and think that the problem is solved. Instead, it hasn’t – and it’s merely been masked.
From a simplistic view, to convey the same power at a higher voltage requires less current, therefore the resistance of the cable causes less voltage and loss. This is true. However, the other half of the story is that Quick Charge is developed to help speed charging of the phone, and instead, it tends to use currents in the 1.2-2A region to best optimize the charging where possible. This is, coincidentally, similar to the currents drawn by regular 5V charging and hence results in a similar amount of voltage drop.
However, people then seem to think that because it runs at a higher voltage, a 0.25V drop isn’t going to be a problem because it’s a smaller percentage of the voltage. This is NOT true, and the reason is simple.
Any voltage dropped over the whole cable assembly’s resistance is produced as heat. This is dependent on current and resistance only, so P=I^2*R. As 5V “regular” charging and Quick Charging nominally operate in similar current regimes, the voltage drop and power lost in the cable is pretty similar.
The two dark green lines indicate the maximum resistance of a single connector and two connectors at end of life. The light-green lines are an estimate of a 50cm 24AWG cable with two connectors, and 1m 24AWG cable with two connectors at end of life. Note that at 1.5A for the cable, expected voltage drop is 0.21 to 0.255V, which is right on borderline acceptable for regular 5V charging. The power dissipated in the cable and connectors is 0.315-0.383W. It’s not an unsubstantial amount – it’s about the same as holding your hand in front of a small battery-operated 3 x COB LED torch.
If you are to expect that 12V charging, being about twice as high of a voltage as 5V, can tolerate twice as much voltage drop, this would increase the power loss to about 0.63-0.766W. This is not a wise move, because the heat has to go somewhere, and now the cable gets twice as much energy dissipated per unit length. Where the cable is well made, the power should be somewhat “evenly” distributed across the cable, but if there is a fault with a contact, the lion’s share of the resistance can concentrate at that point. In the worst case, blindly pulling current despite a falling voltage compounded with a localized resistance in the connector can result in melted connectors and damage to devices. A higher charging voltage does not fix the issue of power being dissipated in and along the cable and connectors!
Practical Effects – A “slow” quick-charge?
If this graph looks a little familiar, it’s because it’s basically the graph from my Mi Power Bank Pro review, just with two traces added to the graph.
In order to test charging current profiles, I grab an appropriate wall-charger and throw a modified charger doctor current shunt in line to measure the current with a Keysight U1461A multimeter connected to a PC.
When first testing the “regular” 2A charger, there was no problem. The unit clocked in a 5 hour and 45 minute charge time. But then I tried to test the QC2.0 charging ability and got the grey curve, clocking in a charge slower than with the non-quick charge adapter.
Thinking this was a compatibility issue, I decided to use a different QC2.0 source, namely the old Mi Power Bank Pro. As a 10,000mAh power bank can’t fully charge a 10,000mAh power bank due to losses in conversion, I took advantage of the simultaneous charging ability. I connected a regular 2A charger to the first power bank to keep it “topping up” while it output QC2.0 to the power bank under test. Because of the close proximity of the output port to the charge port, I fitted a 20cm 24AWG USB extension cable. Interestingly, the charge was still slower than just doing it from a regular 2A charger.
The fault was not with the power bank, as I reverted to the original QC2.0 wall-charger (to be shown in a future posting), but with a small modification.
I noticed that the shunt was not making quite a solid contact with the USB port on the QC2.0 charger only, so I decided to “crimp” the USB socket to flatten it slightly. This increases the contact pressure, which should improve contact resistance. Guess what? It worked and produced the ~3 hour 40 minute curve.
This is a practical illustration of the following points:
- Having quick-charge does not necessarily overcome USB cable and contact resistance as this is a dynamic function of the “mating” of the two connectors. Each plug and unplug can produce slightly different results.
- USB connectors are not made equal – what worked with my Xiaomi 2A charger did not work properly with my QC2.0 wall adapter.
- While a known good source of the old Mi Power Bank Pro was used, the addition of an extension lead added additional contact and cable resistance, nullifying any benefits. As a result, it’s hard to know if you’re improving the situation or not when juggling around cables and chargers.
- While it might be easy to fault the charger doctor’s connector as being “cheap and out-of spec”, chances are, most products have connectors which vary in dimensioning enough to create problems at high currents. The device sensed the resistance and backed-off its current draw appropriately to prevent any damage. However, compared to a proper contact with the 2A charger, quick charging proved to be (ironically) slower prior to this “hack”.
While pinching the connector does improve the contact pressure and contact resistance, it is not advisable to do. This is only a “temporary fix”, as by pinching the connector, you can cause permanent damage to the mated connector. This can happen by “grabbing” the tongue too tightly such that it fractures on applied stress or attempted disconnection, additional fatiguing of the contact springs through over-pressure and wear to the plastic tongue which can cause it to “thin” more rapidly and fail to apply proper pressure to other connectors. It will also cause the plug’s pin’s to be “flattened” more quickly, and the gold plating on both plug and socket to wear rapidly.
Other Issues with USB cables
While I have mentioned cable and contact resistance, there is another element of resistance that can come about from the connection of the wire to the plug itself. This point is usually soldered, and depending on the quality of the cable-strip and solder operation, the resistance can vary. It should be quite small by comparison, however, there has been some evidence to suggest that poor quality connections can occur in cheap cables due to over-stripping of conductors resulting in a few strands being fractured at the strip and not making connection. This produces a localized area of increased resistance, and could be responsible for cases where the connector-plug side shows melting.
Some other cables have been made with non-copper wires as well, which will likely have a higher resistance for the same wire gauge. Be careful!
There are “charge only” cables with only the two power conductors connected, but no data connections. Some may have them locally shorted to present as a dedicated charger to the end device. These cables are incompatible with Quick Charge technology which requires the use of the D+ and D- lines for signalling.
Another issue is with insulation wear-out. As USB cables get flexed quite frequently, areas near the plug may be flexed sufficiently for insulation between wires to break within the outer sheath. This is especially probable for specially “thin” or flat cables where regular PVC insulation is replaced by enamel coating (as with headphone cords). While not directly observable from the outside, such loss of insulation results in an internal short circuit within the cable, which can serve to heat the cable and cause it to burn. I suspect this may be what happened in this “viral” image of a burnt cable on a bed. Regular chargers are generally current-limited to source a total of 5 or 10W, whereas fast-chargers can source up to 18W. This is not insignificant, as a hand-held glue gun or battery-operated soldering iron needs just 7W to operate.
This isn’t as rare as it might seem either – I have been approached by a friend who claimed the Xiaomi power bank I recommended was poor because it charged the phone slowly and kept discharging on its own when connected to the cable with nothing attached. The truth was, the cable had a high-ish resistance internal short which was not enough to cause visible damage, but enough to waste energy. Had the cable worn further, a more drastic result may have eventuated, but the power bank was not at fault.
Conclusion
While the market was quick to respond to the wire gauge problem, it turns out connector resistance is equally as important, or even more important. Problems with connector resistance can arise due to improper connector design, improper dimensions in manufacturing and wear and tear to gold plating on contacts.
Simply upping the voltage may help the symptoms, but does not solve the underlying inefficiency of energy being lost as heat. Where quick-charging is used to improve charge times, the current flow magnitude is roughly the same and there is still a risk that poorly constructed cables with poor contact resistance can heat-up and melt. As a result, even if higher voltages are employed, devices are still sensitive to voltage drops in cabling and connectors to avoid the possibility of excess heat build-up which could lead to fire risk and property damage.
Traditional USB connectors were not specified with close tolerances for dimensions, which results in such problems in ensuring good contact between connectors of different makes. Cost reduction, by reducing materials used, and unconventional USB connector designs also can cause issues. Connectors wear out and become damaged over time. Newer connectors such as USB-C are much tighter on tolerances and should improve the situation, despite the smaller connectors and contact areas.
The solution to the issue is, unfortunately, not so simple for existing “legacy” USB connectors. Most of the time, consumers have absolutely no say about the connectors used in their devices, chargers, cables, etc. and take it for granted that it works well as it’s “USB” standard. Ensuring that products use “name brand” connectors from trusted connector companies (e.g. Amphenol, Molex, Foxconn, Pheonix Contact, etc) that are properly terminated can help. Reducing unnecessary connection/disconnection to avoid wear and avoiding physical strain on the connector is also advised. In a pinch, the situation might be helped by “crimping” the connector slightly, but that is likely to cause accelerated connector wear and is not advised.
Aside from the connector, the wire-to-connector joint is also a potentially vulnerable point, as well as the insulation within the cable assembly. Reports of “phone fires” and “connector melting” often fail to distinguish as to the true cause.
Recommend
About Joyk
Aggregate valuable and interesting links.
Joyk means Joy of geeK