
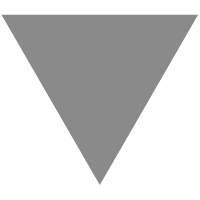
How We Slowed the Subway Down – Home Signal
source link: https://homesignalblog.wordpress.com/2022/12/21/how-we-slowed-the-subway-down/
Go to the source link to view the article. You can view the picture content, updated content and better typesetting reading experience. If the link is broken, please click the button below to view the snapshot at that time.
How We Slowed the Subway Down – Home Signal
Thoughts on transit, urbanism and industry in New York and beyond
Every day, hundreds of thousands of New Yorkers lurch into dusty darkness as their Manhattan-bound 4 or 5 train leaves Brooklyn’s Franklin Avenue station. As the blurred fluorescence of the station cuts to the brown-grey textures of tunnel walls, their trains speed up, and tilt uphill, following the lines of tortured topography bequeathed on Brooklyn by a glacier 18,000 years ago. And then, the train slows down. And then it speeds up. And then, again, it slows down. See-sawing back and forth between fast and slow while screeching around the curve which carries trains onto the broad, downwards-sloping diagonal cut that is Flatbush Avenue, these trains’ path from Franklin to Atlantic Avenue is one whose variability is a story of signaling, speed control, and a subway system locked in a slow-moving crisis.
In 2018, Aaron Gordon, then working at the Village Voice, wrote an article on the subway’s performance woes. The system of 2016-2018 was in crisis; on-time performance had plunged below 75 percent and ridership had begun to fall. Up until that point, most mainstream press coverage had ascribed the system’s reliability struggles to old signals, deferred maintenance, and other, similar stories of disinvestment. What Gordon, and the dozens of articles written on the subject after he published his piece, showed was that the subway’s problems had a lot more to do with the low-level erosion of capacity and speed across the system than they did some meltdown of technology. Years of speed control signal installations, scared operators, and increasingly restrictive operating rules had taken their toll on the system, and as ridership and maintenance loads ticked upwards, the system devolved into a crisis. Important and revealing as this coverage might have been, it did little to illuminate the deeper roots of those signaling decisions. Why did the agency add all these speed restrictions? Why were train crews so scared of moving fast? Why, in other words, did the subway slow down without seemingly anyone working to stop it?

A speed restriction signal (a one-shot grade timer) on the D line in Brooklyn. Image: me
Signals, 101
The subway’s signal system exists to keep riders, crews, and trains safe. As electrified, grade separated rapid transit systems evolved into a category of urban railroading distinct from streetcars during the last quarter of the nineteenth century, signal systems became a key means of organizing and bounding the increasingly speedy movement of trains and people. And, as death tolls rose, accomplishing safety seemingly required these signal systems not only to advise train operators on whether they could proceed safely, but also to enforce those advisements. In that mandate to ensure life and limb lies the origins of the speed crisis.
The working theory of the subway’s signal enforcement begins like this: provided a means of activating the emergency brakes on a train passing a red signal (in New York, a trip stop), trains must be kept far enough apart that a speeding train passing a stop signal will not collide with its leader. That distance varies extensively, dependent as it is on the maximum potential speed at which a train can pass a signal, the slope of the track, and so on – but this commitment to maintaining one ‘safe braking distance’ between trains generally does not.
To achieve that braking safety, the subway’s signal architecture works around the principle of ‘two-block control.’ Every track on the subway is cut up into blocks, electrically isolated sections of line that can detect occupancy by sending current through the axles of passing trains. In two-block control, the signal system keeps at least one unoccupied block – really, however many blocks you need to provide that braking distance with a margin for error – between one train and the next. In signal-ese, the signal’s control line – the distance ahead of it which, if occupied, will make that signal red – extends one safe braking distance, plus one block, ahead of the signal. At junctions, similar rules apply. A switch lined against a train, or a train crossing in front of another is treated by the signals in the same way as is occupied track. So, you can just put these elements together and get safety. Right?

Diagram by me. And yes, I’m simplifying. If you want more detail, see here.
Not so fast. Two-block control is one of those inventions that makes for nice theoretical explanations but horrifically complex practical implementation. To understand why, let’s imagine a short subway line. Our imaginary railroad has four stops, two tracks, an S-curve, and a downhill slope. Remember: the question signal engineers ask themselves is “how far ahead of [this signal] do I need to keep clear so a speeding train can stop before hitting the train in front.” If you treat this question very literally, you might imagine a train that leaves the line’s north terminal and just keeps accelerating. After traversing the downhill across the whole line, it would be moving very fast by the last stop, forcing braking and train separation distances of incredible length. But could it even get to those speeds? The curve in the middle is, let’s say, good for only 25 miles per hour. By that point in its trip, our imaginary train would be cruising along at 60 – which is to say that a signal system for this line designed around constant acceleration would be one which ‘bakes in’ a derailment.

Diagram by me.
To make signaling work, engineers thus needed to handle speed. That meant figuring out ways to enforcing limits, and to deal with long train separation distances. It also meant establishing a set of basic assumptions about the behavior of trains and their crews.
The New York subway uses a type of signal known as a “grade timer” to regulate speed. Its logic is simple. Signal engineers know that a train moving at 50 feet per second (about 34 miles per hour) would take 10 seconds to traverse a 500-foot section of track. So, they installed timers in signals, set to the amount of time a train moving through the block of track in front of the signal should take to pass through it. Timers act as red signals until their countdowns complete, so trains passing such signals are stopped if they passed before the timer completed – which is to say, at a higher-than-desired speed. Later timers would give train crews two tries (“shots”) at getting that speed right, making operations a bit easier on the operators. But in all their forms, these devices allowed engineers two key capabilities: controlling speeds, and thus separation distances, on downhills; and enforcing speeds in areas of risk-laden track. On our imaginary subway line, timers could bridge the gap between assumed constant acceleration and the curve and separation issues that result from it: they would make this route’s service safely possible.

Diagram by me. The timers shown here are one-shots; two shots have more complex signal and max speed math.
Grade timers, however, only got engineers so far. Subway signal systems might exist to keep subways safe, but lest they compromise the effectiveness of the subway service, they also need to provide capacity. A two-block control system designed to stop speeding trains does not always do a great job of that, even if you add lots of signals. Continuing with our example, imagine we put in a signal system that assumes trains make stops at stations. It would have more lenient control lines than even our timer-controlled constant-acceleration design, but would still wind up with a few signals enforcing long train separation distances — for example, the signals with three-block long control lines approaching the rightmost stop.

Diagram by me.
Now, imagine two trains following each other closely into that last stop. When the first train is stopped in a station, the second train is held well back from it, as the signal system protects the leader from a follower travelling at speed. But the follower here is not moving quickly; it is, in fact, not moving at all. Why not let it get closer, provided it keeps to a low speed? Engineers’ response to this once again involved timers: some signals approaching stations and junctions will allow trains to pass if they are moving at a speed slow enough that the next signal has enough space to stop them if they begin to accelerate. These devices are known as “station timers.”

Diagram by me. For a more extensive discussion of station time signals, see here.
Station timers and grade timers are, at the end of the day, instruments. Whether and how they are ever used depends on a set of engineering assumptions, which directly concern the expected behavior of trains and crews, but really are about the limits of socially acceptable risk, and socially desired service. The most basic and immediate one in our example is about whether trains are assumed to stop at stations. But you can (and have to) go beyond that. Can we assume low train frequencies to save on more complex signal arrangements with more signals? Are we willing to assume that our train operators are alert and attentive? Do we design the signal system around that assumption too, baking signed-but-not-enforced speed limits into the assumed speeds of trains passing signals beyond it? What about switches – do we need to enforce trains’ speed over them, or do we assume that operators are paying attention when taking a diverging route? Or, better yet, station time signals. If an operator has their train well enough under control to reduce a trains’ speed to 20 miles per hour and clear a station timer, is it not then possible to rely on their awareness, rather than signal design, to prevent collisions with trains in front?
Eventually, you reach this list’s conclusion, which lies in an unassuming technical question about the trains themselves. After you have figured out what they will look like, and how fast they will run, you need to give signal engineers two facts about them: how fast they can accelerate, and how quickly they can stop. And you need to make sure that your car equipment engineers keep those values the same until you replace the signal system. Remember, the whole point of having signals is keeping a train going at any given location’s maximum possible speed enough braking distance from its leader to prevent a collision. If your gain acceleration performance or lose braking power without rebuilding your signal system, the whole pyramid of assumptions comes crashing down.

In a nutshell, what created the speed crisis on New York’s subway was that engineers and managers at New York City Transit changed almost all of those assumptions ahead of the signal redesign process. When the mess they created was made violently, tragically visible, people freaked out.
Making a Crisis
In 1948, New Yorkers excitedly greeted a new fleet of train cars. As they were barged across New York Harbor, they received a water cannon salute from a fireboat, and were adorned with a massive banner calling out to spectators along the city’s waterfront “New Yorkers: LOOK YOUR NEW SUBWAY CARS.” And indeed, these were special cars. The first new equipment delivered to the system since the ‘30s, they were hailed by advocates and management alike as the first step into a new, better transit system.
Perhaps these cars most important legacies, however, lay between their wheels. Unlike previous subway cars, the R10s were equipped with more powerful motors, giving them starting acceleration rates of 2.5 miles per hour per second, versus 1.75 on older cars. They also had a new braking system, which while mechanically more advanced, was slightly less powerful than those on older cars. The R10s’ predecessors could stop in 230 feet from 30 miles per hour; the R10s and their successors needed 250. It remains unclear how agency engineers weighed these factors when ordering these new cars – New York City Transit did not have a formalized speed policy committee until 1989 – but the outcomes of their choices were dramatic. These changes invalidated the basis of all signal designs preceding the introduction of the R10s. So, while signaling from then onwards would be based on the R10’s performance characteristics, this change began to set the stage for a crisis: every foot of track governed by older signals became a source of risk.
From there on out, conditions only worsened. Through the 1950s and ‘60s, New York City Transit focused on maintaining and incrementally improving its subway system. In some respects, this was good for signaling: older, pre-R10 signal installations were replaced with ones whose design matched the capabilities of the era’s equipment. But in others, it fed the fire.
One of New York City Transit’s key initiatives through the postwar period was platform lengthening. Between the 1940s and early 1970s, the agency extended all stations on the numbered lines to handle 10 car trains (11 on the 7), and converted much of the former BMT to handle 10 cars as well. These projects increased capacity, but they sometimes added to the system’s signal problems. Most subway stations have signals right before and right beyond the platform, so that the platform is contained entirely within one (or is subdivided into multiple) blocks. Lest platform extensions reduce line capacity, New York City Transit’s engineers hoped to preserve that property of station-area signaling, so in areas where the signal system had not been provisioned for longer platforms, or where signal system rebuilds did not accompany platform work, they simply moved signals. The changes involved were small – about 50 feet or so – but they worked to compromise the signal system’s safety. If your blocks and trip arms are not where designers imagined they’d be, and if you don’t take extensive action to remediate that disjunct, your system is less safe.

The final piece of the subway signaling crisis would fall into place twenty-odd years after the last platform extension, at twelve minutes past midnight on August 28, 1991. That night, work crews were doing some track maintenance on the Lexington Avenue Line’s (4/5/6) southbound express track between 14th Street and Brooklyn Bridge, so express trains were crossing onto the local track at the switches just north of Union Square Station. Those crossovers were sharp, good for only about fifteen miles per hour. So, when the intoxicated train operator of a southbound 4 train plowed into them at 50, his train derailed, killing 5 people, and causing so much structural damage to the tunnel that Park Avenue above it sank by half an inch.
The derailment sparked dramatic changes in subway management. The agency began taking drug and alcohol testing very seriously, while beefing up its supervisory ranks. It also shocked the agency into altering its signaling assumptions. Previously, train operators were generally thought to be reliable enough that they would heed speed limits when they approached switches or other areas of sensitive trackwork (the assumption that trains would heed station stops disappeared around 1980 for unknown reasons). After that night, those assumptions were no more: signal designs from then on would assume reckless operation. Having been so violently burned by their past signaling approaches, they would leave less up to chance.
With this policy change, New York City Transit’s signaling transition was complete. By changing assumptions about acceleration, braking and operator awareness, and by altering infrastructure with little regard for signaling impacts, the agency had undermined the technological and institutional underpinnings of signal safety. Each resignaling project brought signals into closer alignment with these assumptions, but those efforts were slow. Transit’s engineers had created a gap between what they thought their signals ought to do, and what they actually did.
Breaking Braking
Lengthening, resignaling, equipment performance changes – these factors all combined to create a system that, by the agency’s standards, was less safe. But while the subway of the later twentieth century was far from a perfect place, the signal system’s problems had yet to become entirely clear. Collisions happened more frequently than they do today, but they generally did not kill. And while there exist news reports of incidents that sound suspiciously like signal system design issues – for example, a B train which rear-ended the system’s revenue collection train in Brooklyn in 1968 – these seem to have been rare. Most of the accidents whose stories made it into the press seemingly had little to do with signal system’s deficiencies.
That began to change in the 1980s, when a series of engineering decisions cast the signal system’s problems in ever-sharper relief. The 1960s and ‘70s had seen New York disinvest from its transit network as the costs of antiurban, racist, and increasingly austerity-minded American policy increasingly fell on New Yorkers’ backs. The resulting transit maintenance crisis defined civic narratives around the subway for years to come – and eventually forced elected leaders to change their approach to the system.
One central element of electeds’ renewal efforts was the creation of regular and fully-funded capital plans. These allowed agency leaders to renew worn infrastructure and equipment, and, most historians agree, helped the transit system on an upwards trajectory into the 21st century. Among the main initiatives funded by those capital plans was known as the General Overhaul Program, or GOH. The idea was simple: the agency would use its capital funds to rebuild its existing fleet of subway cars to address defects and install more reliable mechanical equipment. Among the many changes made as a part of this program was the replacement of brake shoes – the things which push against wheels to stop trains – across the subway fleet. Up until that point, most cars had had shoes made of cast iron, long the standard in the railroad industry. However, in the 1950s, material scientists perfected ceramic composition brake shoes, which allowed smoother, more consistent brake performance at lower brake cylinder pressures. Agency engineers hoped that installing these shoes would help save the agency money, and mitigate the subway’s persistent dust control and noise issues. This change from cast iron to composition shoes was a perfectly sound idea, but somewhere in that process, New York City Transit’s engineers lost the plot, because the changeover inadvertently compromised trains’ brakes.
It took the Union Square derailment for agency management to realize that there was a problem. Operators had been noticing degraded braking performance for years, but before that night, their complaints evidently failed to reach – or interest – agency management. However, only two days after the accident, a group of New York City Transit managers ordered a braking performance test with the same type of cars (R62s) that had been involved in the crash. When crews ran their tests, the cars failed spectacularly, exceeding the signal system’s assumed stopping distances by 100 feet or more. Over the coming months, the agency’s own board of inquiry worked with investigators at the New York State Public Transportation Safety Board to do more braking tests with R62s, all of which yielded the same outcomes. Evidently, in the changeover from cast iron to composition brake shoes, the agency’s car equipment engineers had compromised the efficacy of at least some trains’ brakes.
One might expect that such an alarming mismatch between design and actual performance would yield a concomitantly intense institutional reaction. But in an agency whose internal organization was described by a contemporary investigator as being a “confederation,” rapid action was not forthcoming. Despite the immense and recognized risks of low-performing brakes, the test schedule initially proposed by NYCT’s car equipment department foresaw braking analyses of the whole fleet taking about six years. Only after the intense protests of safety regulators did they agree to shorten their timeline – to four years. And only after another collision, and another spectacularly failed brake test, did the agency’s car equipment bureaucracy agree to quickly run some basic, diagnostic brake tests on the entire fleet. When these wrapped up in February 1993, agency leaders faced the undeniable reality that the subway’s signal system had been compromised: not a single car class performed within design specifications.
The agency’s response to these problems was initially weak. As they grew appreciative of the scale of the crisis, they adopted a much more lenient braking standard (giving 311 feet from 30 mph, versus 250) for the design of future signal systems, and funded a study of signal safety. What they did not do was implement braking system fixes. Their tests had showed that increased brake cylinder pressures would remedy cars’ problems, but through 1994 and 1995, the agency made little effort to implement that change – even on cars that were already cycling through the overhaul process. Later, agency officials would admit they had had “no concrete timetable” on which they planned to fix these safety problems.
Their apathy would prove disastrous.
Around 6 in the morning on June 5, 1995, the day’s morning rush hour was getting started. High above Kent Avenue on the Williamsburg Bridge, riders on an M train waited patiently for a red signal to clear, and for their journey into Manhattan to resume. But after about three minutes of sitting in place, there was a loud crash, and their train leapt forward thirty feet. They had been hit by the J train behind them; fifty-four were injured, and one was dead.

The Williamsburg Bridge crash. By National Transportation Safety Board – NTSB report, Public Domain, https://commons.wikimedia.org/w/index.php?curid=69840209
That crash should not have been possible. Over the coming months, investigators would not only find that the train’s acceleration performance was well in excess of what the 1915-vintage signals on the Williamsburg Bridge had been designed to handle, but also would pull back the curtain on the system’s braking problems, finding that the train’s brakes were wildly out of compliance with signal system assumptions. As this news broke into the press, and as investigators honed in on these braking and signaling issues as root causes of the Williamsburg collision, the agency was forced to take rapid, decisive action.
Timers, Everywhere
New York City Transit’s immediate response to the Williamsburg Bridge crash had three parts. The first two had to do with acceleration and braking performance. The agency did not slow its cars so much that they conformed with pre-1948 car specifications, but they did slow them enough to ensure that they perfectly obeyed the signal system’s designed acceleration curve; in the years between 1948 and 1995, cars had actually gotten even faster. They also, finally, restored brake performance to pre-GOH levels, allowing the agency to safely and honestly return to the 250 foot/30mph braking curve that had governed signal design until the 1993 braking tests.

Acceleration curves showing the pre- and post-reduction speed curves and the signal system’s design limit. From here.
The third and final component entailed modifying the subway’s existing signal system to improve its safety. The acceleration and braking modifications brought train performance back into closer alignment with signal design, but problems remained – around busy stations and complex junctions, in areas with pre-1948 signaling, and otherwise. To provide full protection against collisions at those locations under the agency’s 1990s assumptions, NYCT proposed to do one of three things: lengthen problematic signals’ train separation distances, add grade timers to limit train speeds, or – in an effort that had been underway since the Union Square accident – add specialized speed control signals that would control train speeds over switches.

Over the ensuing fifteen years, the agency would forge ahead with these modifications, identifying and deploying fixes for hundreds of problematic signals. With each of these minor changes, trains slowed down. Rides that were once fifteen minutes became sixteen, then seventeen; stations that once could pump trains out every 100 seconds after a disruption could now only do every 110, 120, or 130. Signal safety improvements eroded the subway system’s capacity, speed, and resiliency: slower trains running further apart do not make for a good trend line.
To some extent, these impacts were just the cost of a safer subway. But the story really is not that simple. After the fervor about the bridge crash died down, and as the agency’s signal improvement efforts turned towards the rollout of Communications Based Train Control on the L line, and other pressing improvement projects, the monies, staff capacity, and track access needed to actually deliver fixes became increasingly scarce. That disinterest had a cost: underfunded signal modification problems stretching over decades of work begat excessively impactful signal designs. Take, for example, a signal whose control line – enforced separation distance – is just a bit too short. Ideally, what you would do to remedy that issue is cut in an insulated joint to define a new block that would bring the control line up to standard. But adding new insulated joints takes money and track time, and if you’re trying to avoid using both of those resources, what you will do instead is simply extend your control line to the next insulated joint – which may be hundreds of feet away. In doing so, you fix the safety problem, but do so at a relatively significant cost to capacity.

But what cost riders the most in this era was New York City Transit’s approach to signal and train maintenance, and operator discipline. In much of the system, the “timer” part of “grade timer” referred to a literal timer relay in a signal cabinet. Electromechanical equipment might be reliable, but it also requires maintenance – to check its function, and also to ensure that track replacement projects and the like do not disrupt signal logic. In the years following the Williamsburg Bridge crash, the agency’s emphasis on speed conservatism permeated maintenance processes, leading to a slow reduction in allowed speeds. A timer that nominally enforced 20 miles per hour might end up clearing at 17, a change that not only aggravated the speed- and capacity-reducing impacts of signal modifications, but also eroded train crews’ trust in the signal system.
That issue of technical trust was only made worse by changes to train cars themselves. Before the Williamsburg Bridge crash, few subway trains had speedometers. The agency expected crews to know their route and their trains well enough that they could work without them. However, pressure from investigating bodies coupled with the conservative speed reduction turn in agency management drove an effort to equip the entire legacy car fleet with speed indicators. Unfortunately, thanks to a combination of poor design choices and poor upkeep, many of these speedometers have proven to be wildly unreliable. So, as train crews navigated the increasingly speed-controlled subway of the aughts, they not only had to build uncertainty around signal design, but equally about the accuracy of their on-train equipment.
What transformed these problems into a much larger crisis was the agency’s commitment to hard-line operational discipline. With their newly expanded supervisory ranks and redoubled focus on stopping red signal overruns, the agency spent the 2000s punishing increasingly large numbers of train operators for operational mistakes. Discouraging reckless operation is an eminently worthy goal, but this trend’s coincidence with declining signal speed reliability served to exponentiate the trust-eroding effects of the agency’s maintenance regime. Given that the agency tended to discount the relevance of mis-timed signals when considering a train crews’ fate following a red signal overrun, operators had every incentive to treat signals cautiously; one’s approach to timers became a litmus test for each train operators’ degree of risk tolerance, and knowledge of their railroad. The upshot of this for riders and planners alike was disastrous. A now-famous analysis of subway signals in 2014 found that the average train crew operated well below design speeds through timers – and it took little more than a bit of attentiveness on your daily travels to notice how widely that ad-hoc safety margin varied between crews.
Hope
Whatever the problems of signal discourse in the 1990s and early 2000s might have been, we today live in the seeming beginnings of a better world. New York City Transit stands alone among North American transit agencies in having a formalized speed improvement program, one whose explicit purpose is to push back against the accreted momentum of the system’s history to raise speeds, restore operators’ trust in their signals and supervisors, and increase ridership. These efforts are not, generally, reverting signal modifications — they rather focus on signed-but-not-enforced ‘civil speed limits’ — but have had large impacts on the speed and reliability of service. For the long run, the agency is also investing billions in signal modernization, replacing the worn signals of our speed crisis with computerized systems – ones which are not only cheaper and more reliable, but also provide the agency with an opportunity to safely break out of the train performance straitjacket imposed by the current signal system. With each increased speed limit, each increment of change to agency discipline culture, each retrained service supervisor, and each new mile of CBTC signaling, the agency is – finally – healing.
But even as the horrors of the subway’s signaling crisis fade into the past, the basic questions which created it will not. A few months ago, the new CEO of Washington’s Metro pointed out the media and regulatory tendency to treat even non-fatal transit incidents as major news stories without batting an eye at the daily carnage on highways. As many pointed out in response to the tweet, there is hardly a one-to-one tradeoff between rail safety and mode share; some of the safest railroads in the globe are also among the most used. But Washington is not going to be Japan tomorrow; New York was not going to become Switzerland between June and December 1995. Obviously transit systems should strive for – and be held accountable to – the highest achievable levels of safety for their mode, but slow, infrequent, unreliable transit pushes people into cars, and into whole new worlds of risk. Alongside all the subway’s lessons about infrastructure’s capacity to encode social values, the way complex institutions negotiate danger, and the importance of treating workers fairly, the signal modification story inevitably begs the question of how infrastructure institutions should navigate known risks. I have no answers here, and I certainly do not think that the American legal or liability assignment system provide good incentives for any parties involved. Every time I ride a Manhattan-bound 5 train through the one-shot timers under Eastern Parkway, I turn the question over in my head. For I know I will have time to give it thought; it is a slow ride to Atlantic.
Recommend
About Joyk
Aggregate valuable and interesting links.
Joyk means Joy of geeK