
3
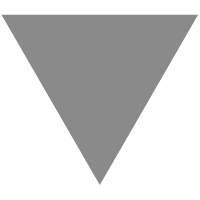
Time-restricted eating with or without low-carbohydrate diet reduces visceral fa...
source link: https://www.cell.com/cell-reports-medicine/fulltext/S2666-3791(22)00332-9
Go to the source link to view the article. You can view the picture content, updated content and better typesetting reading experience. If the link is broken, please click the button below to view the snapshot at that time.
Highlights
- •LCD, 8-h TRE, and their combination significantly reduce body weight and subcutaneous fat
- •TRE yields more benefits on visceral obesity and cardiometabolic outcomes than LCD
- •Combination intervention induces more weight loss compared with LCD or TRE alone
Summary
Overconsumption of carbohydrate-rich food combined with adverse eating patterns contributes to the increasing incidence of metabolic syndrome (MetS) in China. Therefore, we conducted a randomized trial to determine the effects of a low-carbohydrate diet (LCD), an 8-h time-restricted eating (TRE) schedule, and their combination on body weight and abdominal fat area (i.e., primary outcomes) and cardiometabolic outcomes in participants with MetS. Compared with baseline, all 3-month treatments significantly reduce body weight and subcutaneous fat area, but only TRE and combination treatment reduce visceral fat area (VFA), fasting blood glucose, uric acid (UA), and dyslipidemia. Furthermore, compared with changes of LCD, TRE and combination treatment further decrease body weight and VFA, while only combination treatment yields more benefits on glycemic control, UA, and dyslipidemia. In conclusion, without change of physical activity, an 8-h TRE with or without LCD can serve as an effective treatment for MetS (ClinicalTrials.gov: NCT04475822).
Graphical abstract

Introduction
Metabolic syndrome (MetS) is characterized by abdominal obesity, elevated blood pressure, and fasting blood glucose (FBG) as well as atherogenic dyslipidemia with high triglyceride (TG) and low high-density lipoprotein cholesterol (HDL-c) levels,, and it remarkably increases the risk of type 2 diabetes mellitus (T2DM) and cardiovascular diseases (CVD). MetS has long been highly prevalent in Western countries and has steeply increased in the Chinese population over the past decades as well. Abdominal obesity is of central importance in the induction of metabolic dysfunctions including hypertension, hyperglycemia, atherogenic dyslipidemia, and release of proinflammatory cytokines by adipose tissue. Since 2004, the prevalence of general obesity in China has increased 3-fold, and abdominal obesity has increased by more than 50%; concomitantly, a rapid increase in the incidences of T2DM and CVD was also observed., Since even mild body weight reduction can ameliorate metabolic dysfunction, the first line of therapy for MetS comprises lifestyle interventions including aggressive dietary adjustment to reduce body weight., Nevertheless, long-term adherence to lifestyle intervention is always a challenge.
Among dietary interventions, low-carbohydrate diet (LCD) seems more ideal to induce weight loss in overweight individuals compared with low-fat diet,, as LCD generally exerts more rapid weight reduction with a greater loss in body fat and maintenance of lean mass., , LCD restricts carbohydrate consumption to <26% of energy intake (or <130 g carbohydrate/day) and does not contain specific carbohydrates, such as starch and sugar, while containing healthy fats and a moderate protein content.,, , The lower carbohydrate content of LCD is known to reduce insulin secretion, which promotes fat oxidation and lipolysis during negative energy balance., In addition to LCD, time-restricted eating (TRE) has become increasingly popular in recent years for inducing clinically significant weight reduction and ameliorating metabolic disorders., , , , , TRE is defined by intentionally restricting the times during the day when energy is consumed, confining the temporal window of food access to a specified number of hours each day, and fasting (water and tea without sugar or any artificial sweeteners are permitted) for the remainder of the day. Importantly, it is not necessary to monitor caloric intake in any way during the eating window. Chronic circadian disruption can aggravate the risk for components of MetS,, , and TRE maintains a robust daily cycle of eating and fasting to support circadian rhythm., , , One of the most popular regimens of TRE is 8-h TRE (also known as “the 16:8 diet”). There are specific regimens of TRE according to the timing of the eating window, including early TRE (eTRE) that involves eating earlier in the day and late TRE (lTRE) that skips breakfast. Although both LCD and TRE have been demonstrated to be metabolically beneficial,,, whether these 8-h TREs could exert a rapid weight reduction effect comparable to that of LCD in adults with MetS has not been assessed yet.
Despite being an underdeveloped and less prosperous part of the country, the prevalence of abdominal obesity in the Shaanxi province in Northwestern China is close to the national average of 31.5%, and it can thus be regarded as a representative sample of China. In this region, regular eating habits include carbohydrate-rich staple foods, and an eating pattern of consuming three meals a day with late dinner and multiple snacks including a midnight snack is prevalent. In this study, we conducted a 3-month randomized clinical trial (RCT) to determine the effects of an LCD, TRE, and their combination on body weight, fat mass, and cardiometabolic outcomes in adults with MetS in Shaanxi, China. Furthermore, we allowed participants to choose freely between eTRE and lTRE, to keep their social eating pattern. We hypothesized that TRE effectively improves metabolic disease risk parameters without restricting carbohydrate consumption and that combination of TRE with LCD leads to additional metabolic benefits.
Results
Participants
As illustrated in Figure 1, 290 participants were screened for this study, and 121 were excluded because they did not meet the inclusion criteria, had scheduling conflicts, or declined to participate. A total of 169 participants were randomized to receive intervention with LCD (group A; n = 56), TRE (group B; n = 57), or their combination (group C; n = 56), and after dropout of seven individuals, 162 individuals finally participated in the study. All participants met three or more MetS criteria at enrollment, and a minority (n = 62) of participants were on medication. This trial started with a 2-week weight stabilization and was followed by a 3-month intervention. At the end of the 3-month trial, 47 participants completed LCD, 44 completed TRE, and 44 completed their combination intervention. The main reason for dropout was scheduling conflicts. Table 1 and Table S1 show the baseline characteristics of the participants (n = 162). In this trial, we allowed participants to choose freely between two meal-eating windows for participants: 8 a.m. to 4 p.m. (eTRE) and 12 p.m. to 8 p.m. (lTRE), and we compared effects of two meal-eating windows using an exploratory analysis. In the TRE group, 38 participants (m/f 23/15) chose eTRE, and 17 participants (m/f 12/5) chose lTRE. In the combination group, 32 participants (m/f 22/10) chose eTRE, and 20 participants (m/f 15/5) chose lTRE. Table S2 shows the baseline characteristics of the eTRE and lTRE subgroups within the TRE and combination groups. At baseline, there were no significant differences in primary outcomes (i.e., body weight and abdominal fat area) or any secondary outcomes (i.e., body composition, glycemic control, plasma lipids, uric acid [UA], and blood pressure) between groups and subgroups. Table S3 clearly shows that participants receiving LCD or combination intervention had decreased intake of food containing high carbohydrates, such as rice, wheat flour, and pastry. Table S4 and Figure S1 show physical activity and daily step counts of participants, respectively, and demonstrate that participants maintained their usual physical activity throughout the study. Furthermore, participants with or without more than 50% dietary log records during the first 2 weeks of the intervention period showed similar responses to every treatment on primary outcomes after 3 months of intervention (Table S5).
Table 1Baseline characteristics of participants
LCD | TRE | Both | p value | |
---|---|---|---|---|
Male/Female (total) | 30/25 (55) | 35/20 (55) | 37/15 (52) | 0.204 |
Age (years) | 41.3 ± 1.4 | 43.0 ± 1.4 | 39.0 ± 1.2 | 0.106 |
Meal-eating window (hours) | 10.6 ± 0.3 | 10.4 ± 0.3 | 10.7 ± 0.2 | 0.730 |
Daily carbohydrate intake (g) | 324 ± 21 | 348 ± 16 | 361 ± 22 | 0.405 |
Weight (kg) | 84.3 ± 2.2 | 84.7 ± 2.0 | 84.9 ± 1.8 | 0.979 |
BMI (kg/m2) | 29.3 ± 0.5 | 29.6 ±.5 | 29.0 ± 0.5 | 0.711 |
Waist circumference (cm) | 96.1 ± 1.4 | 96.8 ± 1.2 | 94.7 ± 1.0 | 0.457 |
Hip circumference (cm) | 105.1 ± 1.4 | 104.5 ± 0.9 | 103.7 ± 0.9 | 0.645 |
Waist-to-hip ratio (WHR) | 0.92 ± 0.01 | 0.93 ± 0.01 | 0.91 ± 0.01 | 0.212 |
Body fat mass (kg) | 33.9 ± 1.0 | 33.2 ± 0.9 | 32.7 ± 0.9 | 0.651 |
Body muscle mass (kg) | 31.2 ± 1.0 | 31.6 ± 1.0 | 32.0 ± 0.8 | 0.818 |
Subcutaneous fat area (SFA, cm2) | 277 ± 11 | 270 ± 9 | 255 ± 9 | 0.307 |
Visceral fat area (VFA, cm2) | 92 ± 5 | 105 ± 5 | 96 ± 4 | 0.112 |
Hemoglobin A1c (HbA1c, %) | 5.7 (0.6) | 5.6 (0.6) | 5.6 (0.8) | 0.853 |
Fasting blood glucose (mmol/L) | 5.10 (0.97) | 5.05 (0.89) | 5.07 (1.08) | 0.763 |
Fasting insulin (mIU/L) | 27.4 (24.7) | 31.8 (24.8) | 28.2 (17.7) | 0.459 |
C-peptide (pg/mL) | 1,608.8 ± 104.2 | 1,660.2 ± 100.1 | 1,651.7 ± 88.5 | 0.923 |
HOMA-IR | 6.76 (9.68) | 7.38 (5.90) | 7.04 (6.67) | 0.612 |
HOMA-IS | 0.17 (0.17) | 0.17 (0.19) | 0.16 (0.12) | 0.473 |
QUICKI | 0.30 (0.04) | 0.29 (0.03) | 0.29 (0.03) | 0.253 |
Uric acid (UA, μmol/L) | 380 ± 13 | 384 ± 13 | 416 ± 16 | 0.144 |
Total cholesterol (mmol/L) | 4.72 ± 0.14 | 4.76 ± 0.13 | 4.73 ± 0.13 | 0.978 |
LDL-c (mmol/L) | 2.99 ± 0.13 | 3.01 ± 0.12 | 3.03 ± 0.12 | 0.974 |
Triglycerides (TG, mmol/L) | 1.74 (1.52) | 2.10 (1.55) | 2.12 (2.56) | 0.086 |
HDL-c (mmol/L) | 1.13 ± 0.03 | 1.10 ± 0.03 | 1.04 ± 0.03 | 0.161 |
TG/HDL-c | 1.58 (1.52) | 1.84 (1.88) | 2.02 (2.87) | 0.044 |
Systolic blood pressure (mmHg) | 130 ± 2 | 136 ± 2 | 131 ± 2 | 0.086 |
Diastolic blood pressure (mmHg) | 82 ± 2 | 87 ± 2 | 84 ± 2 | 0.112 |
LCD, low-carbohydrate diet; TRE, time-restricted eating; Both, combination treatment; BMI, body mass index; HOMA-IR, homeostasis model assessment insulin resistance; HOMA-IS, homeostatic model assessment of insulin sensitivity; QUICKI, quantitative insulin-sensitivity check index; LDL-c, low-density lipoprotein cholesterol; HDL-c, high-density lipoprotein cholesterol. All data are presented as the mean ± standard error of the mean (SEM) for normal distribution or median (interquartile range) for abnormal distribution. Differences between treatment arms (LCD, TRE, and both) were tested by one-way ANOVA or Kruskal-Wallis H test.
LCD, TRE, and their combination reduce body weight in adults with MetS
As compared with baseline, after 3 months of intervention a significant reduction of body weight was observed in all three groups (Figures 2A and 2B ), and only combination treatment induced a further reduction of body weight at month 3 compared with month 2 (Figure 2C and Table 2). Moreover, as shown in Table 2, combination treatment induced a higher reduction in body weight (−5.0 ± 0.4 kg) compared with either LCD (−2.2 ± 0.3 kg, p < 0.001) or TRE alone (−3.4 ± 0.4 kg, p = 0.004), and a significant difference in body weight reduction was also observed between LCD and TRE treatment (p = 0.013). Furthermore, both eTRE and lTRE alone or combined with LCD led to a sustained reduction of body weight as early as after 1 month, which persisted over 3 months (Table S6).
Table 2Change in body composition and metabolic risk factors after 3 months intervention among participants
LCD | p value | TRE | p value | Both | p value | p value for pairwise comparison | ||||
---|---|---|---|---|---|---|---|---|---|---|
N = 55 | N = 55 | N = 52 | LCD vs. TRE | LCD vs. Both | TRE vs. Both | |||||
Days of adherence (days) | – | 55.5 ± 3.5 | – | 65.9 ± 3.0 | – | 57.7 ± 3.1 | – | 0.024 | 0.631 | 0.059 |
Willingness to continue the diet (n/total, %) | – | 46/47 (98) | – | 43/44 (98) | – | 36/44 (82) | – | 0.962 | 0.010 | 0.014 |
Meal-eating window (hours) | Follow-up | 10.0 ± 0.3 | – | 6.5 ± 0.3 | – | 6.8 ± 0.3 | – | – | – | – |
△ | −0.6 ± 0.3 | 0.075 | −3.9 ± 0.4 | < 0.001 | −3.9 ± 0.4 | < 0.001 | < 0.001 | < 0.001 | 0.992 | |
Daily carbohydrate intake (g) | Follow-up | 149 ± 12 | – | 327 ± 15 | – | 140 ± 11 | – | – | – | – |
△ | −175 ± 22 | < 0.001 | −21 ± 14 | 0.137 | −221 ± 20 | < 0.001 | < 0.001 | 0.125 | < 0.001 | |
Weight a (kg) | 1 M Follow-up | 83.0 ± 2.2 | – | 82.6 ± 2.1 | – | 82.1 ± 1.8 | – | – | – | – |
1 M △ | −1.7 ± 0.3 | < 0.001 | −2.4 ± 0.4 | < 0.001 | −2.6 ± 0.4 | < 0.001 | 0.116 | 0.082 | 0.768 | |
2 M Follow-up | 82.2 ± 2.2 | – | 81.4 ± 2.0 | – | 80.5 ± 1.8 | – | – | – | – | |
2 M △ | −2.5 ± 0.3 | < 0.001 | −3.7 ± 0.4 | < 0.001 | −4.2 ± 0.4 | < 0.001 | 0.015 | 0.002 | 0.347 | |
3 M Follow-up | 82.3 ± 2.4 | – | 81.2 ± 2.2 | – | 80.2 ± 1.8 | – | – | – | – | |
3 M △ | −2.2 ± 0.3 | 0.213 | −3.4 ± 0.4 | 0.323 | −5.0 ± 0.4 | 0.028 | 0.013 | <0.001 | 0.004 | |
BMI (kg/m2) | Follow-up | 28.3 ± 0.4 | – | 28.1 ± 0.4 | – | 27.2 ± 0.4 | – | – | – | – |
△ | −0.9 ± 0.2 | < 0.001 | −1.4 ± 0.3 | < 0.001 | −1.8 ± 0.2 | < 0.001 | 0.098 | 0.003 | 0.280 | |
Waist circumference (cm) | Follow-up | 93.6 ± 1.6 | – | 92.7 ± 1.5 | – | 91.4 ± 1.4 | – | – | – | – |
△ | −2.4 ± 1.1 | 0.035 | −4.2 ± 1.0 | < 0.001 | −3.3 ± 1.2 | 0.008 | 0.248 | 0.603 | 0.563 | |
Hip circumference (cm) | Follow-up | 102.3 ± 0.9 | – | 103.0 ± 0.9 | – | 100.9 ± 1.0 | – | – | – | – |
△ | −2.7 ± 0.8 | 0.001 | −1.5 ± 0.7 | 0.046 | −2.8 ± 0.9 | 0.002 | 0.263 | 0.945 | 0.267 | |
Waist-to-hip ratio (WHR) | Follow-up | 0.91 ± 0.01 | – | 0.90 ± 0.01 | – | 0.90 ± 0.01 | – | – | – | – |
△ | −0.01 ± 0.01 | 0.421 | −0.04 ± 0.01 | < 0.001 | −0.01 ± 0.01 | 0.493 | 0.023 | 0.994 | 0.033 | |
Body fat mass (kg) | Follow-up | 31.9 ± 0.9 | – | 31.9 ± 0.9 | – | 29.8 ± 0.9 | – | – | – | – |
△ | −2.0 ± 0.4 | < 0.001 | −1.3 ± 0.6 | 0.028 | −3.0 ± 0.5 | < 0.001 | 0.301 | 0.103 | 0.041 | |
Body muscle mass (kg) | Follow-up | 31.3 ± 1.0 | – | 31.1 ± 0.9 | – | 31.5 ± 0.8 | – | – | – | – |
△ | 0.1 ± 0.2 | 0.524 | −0.5 ± 0.3 | 0.046 | −0.5 ± 0.2 | 0.064 | 0.048 | 0.064 | 0.893 | |
Subcutaneous fat area (SFA, cm2) | Follow-up | 253 ± 12 | – | 245 ± 10 | – | 231 ± 10 | – | – | – | – |
△ | −23 ± 5 | < 0.001 | −24 ± 8 | 0.003 | −24 ± 8 | 0.006 | 0.927 | 0.988 | 0.949 | |
Visceral fat area (VFA, cm2) | Follow-up | 98 ± 6 | – | 92 ± 5 | – | 86 ± 4 | – | – | – | – |
△ | 6 ± 5 | 0.277 | −13 ± 5 | 0.008 | −10 ± 3 | 0.006 | 0.009 | 0.016 | 0.548 | |
Hemoglobin A1c (HbA1c, %) | Follow-up | 5.7 (0.6) | – | 5.6 (0.6) | – | 5.6 (0.7) | – | – | – | – |
△ | 0.0 (0.3) | 0.404 | 0.0 (0.3) | 0.385 | −0.1 (0.4) | 0.021 | 0.928 | 0.126 | 0.157 | |
Fasting blood glucose (mmol/L) | Follow-up | 5.22 (1.11) | – | 4.76 (1.01) | – | 5.01 (1.23) | – | – | – | – |
△ | 0.07 (0.81) | 0.820 | −0.18 (0.65) | 0.024 | −0.21 (0.96) | 0.048 | 0.102 | 0.113 | 0.739 | |
Fasting insulin (mIU/L) | Follow-up | 23.7 (19.4) | – | 26.5 (20.3) | – | 18.2 (20.8) | – | – | – | – |
△ | −3.1 (10.4) | < 0.001 | −3.3 (12.7) | < 0.001 | −5.5 (14.4) | < 0.001 | 0.394 | 0.319 | 0.781 | |
C-peptide (pg/mL) | Follow-up | 1,424.1 ± 85.2 | – | 1,416.3 ± 80.3 | – | 1,332.7 ± 71.0 | – | – | – | – |
△ | −184.6 ± 47.6 | < 0.001 | −243.9 ± 66.0 | 0.001 | −319.1 ± 67.8 | < 0.001 | 0.468 | 0.104 | 0.429 | |
HOMA-IR | Follow-up | 4.64 (4.70) | – | 5.73 (4.39) | – | 4.17 (4.41) | – | – | – | – |
△ | −1.15 (2.99) | < 0.001 | −1.04 (4.53) | < 0.001 | −2.16 (4.82) | < 0.001 | 0.427 | 0.049 | 0.258 | |
HOMA-IS | Follow-up | 0.23 (0.29) | – | 0.22 (0.42) | – | 0.29 (0.21) | – | – | – | – |
△ | 0.03 (0.12) | < 0.001 | 0.04 (0.25) | < 0.001 | 0.10 (0.20) | < 0.001 | 0.421 | 0.042 | 0.245 | |
QUICKI | Follow-up | 0.31 (0.04) | – | 0.30 (0.04) | – | 0.31 (0.04) | – | – | – | – |
△ | 0.01 (0.02) | 0.001 | 0.01 (0.03) | < 0.001 | 0.02 (0.03) | < 0.001 | 0.144 | 0.004 | 0.157 | |
Uric acid (UA, μmol/L) | Follow-up | 363 ± 14 | – | 345 ± 12 | – | 364 ± 12 | – | – | – | – |
△ | −17 ± 11 | 0.125 | −40 ± 9 | 0.001 | −51 ± 13 | < 0.001 | 0.146 | 0.039 | 0.259 | |
Total cholesterol (mmol/L) | Follow-up | 4.91 ± 0.15 | – | 4.79 ± 0.14 | – | 4.87 ± 0.15 | – | – | – | – |
△ | 0.19 ± 0.12 | 0.112 | 0.03 ± 0.17 | 0.866 | 0.14 ± 0.13 | 0.289 | 0.432 | 0.777 | 0.603 | |
LDL-c (mmol/L) | Follow-up | 3.27 ± 0.14 | – | 3.14 ± 0.14 | – | 3.33 ± 0.15 | – | – | – | – |
△ | 0.28 ± 0.13 | 0.042 | 0.13 ± 0.14 | 0.343 | 0.30 ± 0.13 | 0.026 | 0.447 | 0.929 | 0.389 | |
Triglycerides (TG, mmol/L) | Follow-up | 1.30 (0.94) | – | 1.60 (1.64) | – | 1.40 (1.59) | – | – | – | – |
△ | −0.15 (1.20) | 0.052 | −0.30 (1.36) | 0.006 | −0.51 (2.01) | < 0.001 | 0.363 | 0.011 | 0.160 | |
HDL-c (mmol/L) | Follow-up | 1.16 ± 0.03 | – | 1.13 ± 0.03 | – | 1.13 ± 0.03 | – | – | – | – |
△ | 0.03 ± 0.03 | 0.288 | 0.02 ± 0.03 | 0.442 | 0.09 ± 0.02 | < 0.001 | 0.869 | 0.136 | 0.109 | |
TG/HDL-c | Follow-up | 1.20 (1.20) | – | 1.49 (1.54) | – | 1.30 (1.33) | – | – | – | – |
△ | −0.02 (1.20) | 0.244 | −0.30 (1.59) | 0.024 | −0.59 (2.13) | < 0.001 | 0.265 | 0.003 | 0.094 | |
Systolic blood pressure (mmHg) | Follow-up | 130 ± 3 | – | 137 ± 2 | – | 131 ± 2 | – | – | – | – |
△ | 1 ± 2 | 0.770 | 1 ± 2 | 0.635 | 1 ± 2 | 0.719 | 0.923 | 0.979 | 0.914 | |
Diastolic blood pressure (mmHg) | Follow-up | 81 ± 2 | – | 85 ± 2 | – | 80 ± 2 | – | – | – | – |
△ | −1 ± 1 | 0.313 | −2 ± 1 | 0.144 | −5 ± 2 | 0.005 | 0.823 | 0.140 | 0.178 |
LCD, low-carbohydrate diet; TRE, time-restricted eating; Both, combination treatment; BMI, body mass index; HOMA-IR, homeostasis model assessment insulin resistance; HOMA-IS, homeostatic model assessment of insulin sensitivity; QUICKI, quantitative insulin-sensitivity check index; LDL-c, low-density lipoprotein cholesterol; HDL-c, high-density lipoprotein cholesterol. All data were presented as mean ± standard error of the mean (SEM) for normally distributed variables or the median (interquartile range) for abnormal distribution. Change scores from baseline were represented by “Δ” in the table. Analyses were conducted using all participants (intention-to-treat), using a linear mixed model with randomized dietary intervention as factor to correct for the correlations of repeated measurements on changes in body weight, and using a multiple imputation approach for other missing data. After 3 months of intervention, pairwise comparisons of change scores between the groups (e.g., TRE vs. LCD, TRE vs. Both, LCD vs. Both) were evaluated by t test or Mann-Whitney U test. For weight a: significant differences compared with 1 month before (paired t test); for other parameters: significant differences compared with baseline (paired t test or paired Wilcoxon test).
TRE, LCD, and their combination reduce subcutaneous fat, while only TRE with and without LCD reduces abdominal visceral fat
Abdominal fat is a pivotal risk factor and one of the drivers of the metabolic risk related to overweight and obesity. Waist-to-hip ratio (WHR), an indicator of abdominal obesity, is more closely correlated to MetS than body mass index (BMI)., As compared with baseline, all three treatments induced a significant reduction of waist circumference, hip circumference, and body fat mass (Figures 2D, 2E, and 2F) after 3 months of intervention. Nevertheless, only TRE induced a more prominent reduction of WHR (−0.04 ± 0.01, Figure 2G and Table 2) compared with LCD (−0.01 ± 0.01, p = 0.023) and combination (−0.01 ± 0.01, p = 0.033), suggesting that TRE more effectively alleviates abdominal obesity than LCD.
Both abdominal visceral fat area (VFA) and abdominal subcutaneous fat area (SFA) play important but distinct roles in metabolic function. Thus, we further dissected the changes in these two fat depots using bioelectrical impedance analysis. Although after 3 months of intervention all three treatments induced similar reduction of SFA (Figure 2H), VFA was only decreased by TRE (−13 ± 5 cm2) and combination treatment (−10 ± 3 cm2, Figure 2I and Table 2). Compared with LCD (6 ± 5 cm2), VFA was significantly reduced by both TRE (p = 0.009) and combination treatment (p = 0.016). Furthermore, as shown in Table S6, eTRE significantly reduced VFA and SFA, whereas lTRE did not, albeit that the change of VFA or SFA induced by eTRE and lTRE alone or combined with LCD did not differ.
TRE with and without LCD improves glycemic control
We next compared the effects of LCD, TRE, and their combination on glycemic control. In line with findings on abdominal VFA, TRE with or without LCD, but not LCD alone, significantly improved FBG and UA (Figures 3A and 3B and Table 2). Only combination treatment significantly decreased hemoglobin A1c (HbA1c) (Figure 3C and Table 2). In contrast, compared with baseline, all three treatments clearly improved fasting insulin levels (Figure 3D), C-peptide, homeostasis model assessment of insulin resistance (HOMA-IR), homeostatic model assessment of insulin sensitivity (HOMA-IS) (Figure 3E), and quantitative insulin-sensitivity check index (QUICKI) (Table 2). Notably, combination treatment caused more prominent changes on UA (combination: −51 ± 13 μmol/L, versus LCD: −17 ± 11 μmol/L, p = 0.039), HOMA-IR (combination: −2.16 [4.82], versus LCD: −1.15 [2.99], p = 0.049), HOMA-IS (combination: 0.10 [0.20], versus LCD: 0.03 [0.12], p = 0.042) and QUICKI (combination: 0.02 [0.03], versus LCD: 0.01 [0.02], p = 0.004) compared with LCD (Figures 3B and 3E and Table 2), indicating that the combination of LCD and TRE is most effective to combat cardiometabolic risk factors among the three interventions. Furthermore, we did not observe any significant differences in parameters related to glucose and insulin metabolism between eTRE and lTRE, whereas combined with LCD, eTRE displayed a further improvement of HOMA-IS (Table S6). As shown in Figures S2A and S2B, HOMA-IS and UA were significantly correlated with VFA but not with SFA.
TRE with and without LCD, but not LCD alone, improves dyslipidemia
LCD did not cause any significant differences in plasma levels of TG, HDL-c, or the ratio between TG and HDL-c (TG/HDL-c ratio) after 3 months of intervention (Figures 3F, 3G, and 3H and Table 2). In marked contrast, TRE with and without LCD significantly reduced TG level and TG/HDL-c ratio, and the change in TG and TG/HDL-c ratio was significantly different between LCD and combination treatment (TG: −0.15 [1.20] mmol/L versus −0.51 [2.01] mmol/L, p = 0.011; TG/HDL-c: −0.02 [1.20] versus −0.59 [2.13], p = 0.003). While TRE did not affect low-density lipoprotein cholesterol (LDL-c) levels, LCD with and without TRE even significantly increased LDL-c levels (Table 2). Moreover, we did not find any significant difference between eTRE and lTRE in the improvements of dyslipidemia (Table S6). In line with the prominent contribution of VFA to glycemic control, TG/HDL-c ratio was only significantly correlated with VFA, not SFA (Figure S2C). Taken together, while LCD adversely affects LDL-c, TRE improves the lipoprotein profile.
TRE with and without LCD, but not LCD alone, reduces diastolic blood pressure
Although none of the treatments had benefits on systolic blood pressure (SBP) (Figure 3I), diastolic blood pressure (DBP) was significantly reduced by combination treatment, but not by LCD and TRE alone (Figure 3J) after 3 months of intervention. Compared with changes of each group, no significant difference in DBP was observed among three treatments (Table 2). When combined with LCD, eTRE significantly reduced DBP, whereas lTRE did not (Table S3), albeit that eTRE did not induce a significantly different effect on DBP compared with lTRE. Both SBP and DBP strongly correlated to VFA but not SFA (Figure S2D).
Adverse events
No serious adverse events were observed. Approximately five adverse events were regarded as probably associated with the diet interventions, including constipation, dizziness, insomnia, dry mouth, and alopecia. The occurrence rate of adverse events was not significantly different among the three groups (Table 3). Independent of the diet intervention, two participants reported the exacerbation of lumbar disc herniation and lithangiuria requiring surgery during the 3-month intervention, which caused withdrawal from the trial.
Table 3Adverse effects among participants
LCD | TRE | Both | p value | |
---|---|---|---|---|
N = 55 | N = 55 | N = 52 | ||
Adverse effects (number, n%) | – | – | – | 0.232 |
Constipation | 0 (0.0) | 1 (1.8) | 3 (5.8) | – |
Dizziness | 0 (0.0) | 1 (1.8) | 2 (5.8) | – |
Insomnia | 3 (5.5) | 0 (0.0) | 1 (1.9) | – |
Dry mouth | 1 (1.8) | 0 (0.0) | 1 (1.9) | – |
Alopecia | 0 (0.0) | 0 (0.0) | 1 (1.9) | – |
LCD, low-carbohydrate diet; TRE, time-restricted eating; Both, combination treatment. Differences between treatment arms (LCD, TRE, and Both) were tested by Chi-square test.
Feasibility and acceptability
We also analyzed acceptability and feasibility of the interventions. As shown in Table 2, participants’ self-reported compliance with their meal-eating window during the 8-h TRE intervention was on average 65.9 ± 3.0 days out of the 3-month intervention period, which was significantly more to adherence to LCD (55.5 ± 3.5 days; p = 0.024). In addition, adherence to eTRE was substantially less (61.4 ± 4.0 days) compared with lTRE (74.9 ± 2.7 days; p = 0.031, Table S6). Nonetheless, at the end of our study, 46 out of 47 participants (98%) in the LCD group and 43 out of 44 participants (98%) in the TRE group who completed the intervention period reported to be willing to continue. In contrast, only 36 out of 44 participants (82%) in the combination group reported to be willing to continue with the intervention, which was significantly lower compared with LCD (p = 0.010) and TRE (p = 0.014).
Discussion
To our knowledge, this is the first clinical trial that directly compared the efficacy of weight loss and improvement of metabolic parameters of an LCD, 8-h TRE, and their combination in adults with MetS. We showed that although all three treatments significantly reduce body weight accompanied by a reduction in SFA, TRE yielded more benefits on abdominal visceral obesity and cardiometabolic outcomes and caused higher adherence to intervention compared with LCD. Moreover, both meal-eating windows of TRE (i.e., eTRE and lTRE) showed comparable beneficial effects on body weight, abdominal visceral fat, glucose metabolism, lipoprotein profile and blood pressure, as well as adherence. In addition, we observed that VFA, but not SFA, significantly correlated with several cardiometabolic parameters, including HOMA-IS, UA, the TG/HDL-c ratio, SBP, and DBP.
In this study, we have followed the ADA recommendation on the LCD, restricting subjects’ carbohydrate intake to <130 g/day and demonstrated a slight but significant reduction in body weight (−2.2 kg; −2.7%) in adults with MetS over the course of 3 months without apparent adverse effects. Similarly, a previous clinical trial showed that LCD treatment of T2DM patients, i.e., 130 g/day carbohydrates without other specific restrictions, caused 1.6 kg body weight loss over 6 months A 12-week randomized study also showed that LCD (<100 g carbohydrates/day) reduced body weight in type 1 diabetes subjects by 2.0 kg. In addition, the beneficial effects of very-low-carbohydrate ketogenic diets (VLCKD; < 50 g carbohydrates/day) on body weight reduction have been assessed.,,, Samaha et al. showed that severely obese subjects with MetS significantly lost body weight (−5.8 kg) after 6 months on a VLCKD (<30 g carbohydrates/day). Another 1-year clinical trial showed that a VLCKD (<40 g carbohydrates/day) intervention resulted in significant weight loss (−3.5 kg) in obese individuals without T2DM or CVD. However, the efficacy and safety of VLCKD and adherence during long-term intervention are still under debate. Besides, we found participants from Northwestern China showed a 10.6-h baseline meal-eating window, which was calculated by participants’ self-report of average three meal times on 2-week recall. This baseline meal-eating window is comparable with the finding from a recent 1-year RCT study in Southern China, which was 10.4-h baseline eating window that was calculated by daily dietary log, food photograph, and eating time. We further demonstrated that an 8-h TRE significantly reduces body weight in adults with MetS (−4.0%), independent of timing of TRE. Several previous clinical trials have evaluated the weight-reduction efficiency of TRE in individuals with MetS.,, Wilkinson et al. found that a 10-h TRE led to an approximately 3% weight reduction and improvements in cardiovascular risk parameters in individuals with MetS. A recent trial showed that 8-h TRE decreased body weight of obese individuals by 2.6% after 3 months Nonetheless, in our study only the combination of LCD and TRE produced clinically significant weight loss, i.e., a reduction of 5.8% from baseline over 3 months.
Our results showed that LCD decreased SFA without affecting VFA, while TRE and the combination treatment decreased SFA as well as VFA. Accumulating evidence indicates that visceral fat is crucially associated with many aspects of MetS, including hypertension, dyslipidemia, glucose intolerance, and insulin resistance, and it is more closely linked to inflammatory and oxidative stress biomarkers than subcutaneous fat., , Our results suggest that compared with LCD, TRE might yield more benefits on cardiometabolic outcomes in adults with MetS. Indeed, in our study, LCD intervention did not significantly decrease FBG levels but prominently reduced insulin levels and ameliorated insulin sensitivity, which are consistent with previous trials,, suggesting that LCD is more effective in lowering blood insulin levels and improving insulin sensitivity than in lowering blood glucose levels. This is likely explained by the fact that most studies were conducted with relatively healthy or overweight individuals but not individuals with T2DM, and not all participants in these studies had elevated FBG levels. In contrast, in our study, TRE intervention improved insulin levels as well as blood glucose levels, and furthermore, the combination of LCD and TRE significantly reduced fasting glucose, insulin, and HbA1c levels in MetS patients. In addition, compared with baseline, TRE with and without LCD reduced UA levels, while compared with changes among treatments, combination treatment caused more prominent reduction on UA. High UA is a strong and independent predictor of MetS and is associated with impaired fasting glucose and insulin resistance., , A recent 6-h TRE trial in overweight individuals with prediabetes revealed an improvement in insulin sensitivity and β cell responsiveness but no reduction in FBG. Fasting might improve glycemic control as a result of metabolic switch from liver-derived glucose to adipose cell-derived ketones, occurring when switching from a fed to a fasted state, and it might induce ketoplasia, decrease fat accumulation, and increase insulin sensitivity.,, However, further studies need to be performed in participants with elevated FBG, prediabetes, or T2DM to better define the effects of LCD versus TRE on glucose regulation. Whether TRE with or without LCD has independent effects on visceral fat and metabolic outcomes or is simply an epiphenomenon of greater weight loss could not be addressed in this study. It is noted that a previous RCT indicated that TRE that induced mild body weight reduction (∼3%) without changing visceral fat mass was accompanied with improvements of insulin resistance and oxidative stress, while another study showed that although there were no effects on body weight reduction, TRE could still improve those cardiometabolic parameters in prediabetic men.
The effects of LCD and TRE on dyslipidemia are highly variable between studies.,,, , , , We observed that LCD alone and combined with TRE adversely increased LDL-c after 3-month intervention, which is consistent with several studies showing that LCD increases cholesterol levels within the large LDL subfractions., In this study, while TRE treatment did not impact HDL-c, TRE and combination treatment, but not LCD, significantly reduced plasma TG levels. In fact, TRE was generally reported not to affect HDL-c, although one study reported a minor improvement. Yet, the effects of TRE on TG levels are still controversial. For instance, some TRE studies demonstrated a reduction in TG,, whereas others showed no significant effects.,,, In addition, we observed that TRE and combination treatment reduced the TG/HDL-c ratio, which could be partly due to reduced VFA by these treatments, but not by LCD alone. Indeed, VFA but not SFA strongly correlates with the TG/HDL-c ratio (Figure S2C). The TG/HDL-c ratio is a well-known predictor for CVD. A reduced TG/HDL-c ratio may be attributed to decreased cholesteryl ester transfer protein (CETP) activity, as CETP mediates the net transfer of CE from HDL to TG-rich lipoproteins in exchange for TG. Previous studies have demonstrated that weight loss induced by a very low calorie diet was correlated with reduced CETP concentration, and CETP inhibition was associated with the improvement of visceral fat. Therefore, TRE, with or without restricted carbohydrate consumption, could significantly improve visceral obesity and reduce TG level and TG/HDL-c ratio, as well as decrease CETP concentration.
In addition, only combination intervention significantly decreased blood pressure in our study. Generally, moderate (5%–10%) weight loss caused by interventions is expected to lead to larger reductions in SBP of 5 mmHg and DBP of 3 mmHg than that of mild (0–5%) weight loss over 6–12 months as shown in a systematic review and meta-analysis. We observed that the mean reduction in DBP (5 mmHg) by combination intervention that produced a moderate weight loss of 5.8% was apparently higher and not accompanied by a reduction in SBP. It should be noted though that our study was not properly powered to observe a significant change in blood pressure, and larger studies are obviously needed to further address the effects of LCD and TRE on blood pressure in MetS patients.
In this study, eTRE shows greater effects on reducing abdominal fat area (both SFA and VFA) than lTRE, while eTRE and lTRE showed comparable benefits on body weight, glycemic control, dyslipidemia, and blood pressure. Nevertheless, participants were not randomly assigned to eTRE or lTRE, and sample sizes were relatively small, so the comparison of eTRE and lTRE was exploratory. Previous studies showed that both eTRE and lTRE improved multiple indicators of cardiovascular health. Sutton et al. conducted a 5-week study comparing eTRE (6-h eating window before 3:00 p.m.) with a control condition (conventional 12-h eating window) and found better glycemic control and improvement of blood pressure by eTRE without significant body weight changes. Furthermore, Cienfuegos et al. found that both 4- and 6-h lTRE caused mild body weight reduction (∼3%) over 2 months when compared with the control. However, several studies on lTRE demonstrated conflicting results regarding body weight., Moreover, the thermic effect of food, insulin sensitivity, and β cell function is better in early morning than night, , because the body is optimized to ingesting food in early morning., , , Thus, an 8 a.m. to 4 p.m. eating window may be applied as a more effective intervention to improve insulin sensitivity. Besides, lipids were also affected by meal timing, which might be due to an increase of fat oxidation in eTRE. However, for participants who find it easier to skip breakfast than dinner, the latter being a more social meal in most cultures, a 12 p.m. to 8 p.m. eating window is an alternative. Thus, it is important to consider participants’ individual schedule and personal preference and allow them to choose the suitable TRE eating window in order to increase efficacy and adherence.
Conclusions
In conclusion, compared with baseline, all three treatments after a 3-month intervention reduce body weight and SFA, as well as some cardiometabolic outcomes, including fasting insulin, C-peptide, and insulin sensitivity index, but only TRE, with and without LCD, significantly reduces abdominal visceral fat, FBG, UA, TG, and TG/HDL-c ratio. More importantly, compared with changes of LCD, TRE and combination treatment further decrease body weight and VFA. Taken together, without changing physical activity, TRE with and without LCD significantly improves glycemic control, atherogenic dyslipidemia, and UA, thus largely improves metabolic disease risk, with TRE being superior over LCD with respect to reducing body weight and abdominal visceral obesity. Therefore, we anticipate that an 8-h TRE without and with LCD can serve as an effective intervention for MetS.
Limitations of the study
First, as is the case for all self-reported dietary intake data and because the daily dietary log was not compulsory, we cannot verify that the data reported by participants represent a complete record of their diet and asking participants to report adherence is subject to recall bias. In addition, the meal-eating window was calculated by participants' self-report of average meal times on 2-week recall without accounting for caloric consumption outside of three meals a day, so this method did not account for day-to-day variation of caloric consumption, and participants’ true eating window is likely being underestimated. Second, except for the combination treatment, both the LCD and TRE treatments did not induce clinically significant weight reduction over 3 months. Longer-term trials are necessary to investigate whether LCD and TRE treatment alone can indeed produce 5% weight loss and lasting benefits to overall health. Third, the comparison of eTRE and lTRE was exploratory as participants were not randomly assigned to eTRE or lTRE and sample sizes were relatively small. Moreover, there were significantly more adherent days in the TRE group compared with LCD, and the absence of a control group is another limitation of this study. Last, only Chinese people living in the Shaanxi province were enrolled in this study, which warrants future validation of our findings in other race or ethnic groups.
STAR★Methods
Key resources table
REAGENT or RESOURCE | SOURCE | IDENTIFIER |
---|---|---|
Critical commercial assays | ||
Human Metabolic Hormone Magnetic Bead Panel | Merck Millipore | Cat#HMHEMAG-34K |
Software and algorithms | ||
GraphPad Prism version 8.0.2 | GraphPad Software | https://www.graphpad.com/ |
R version 4.1.3 | R-Project | https://cran.r-project.org/ |
Other | ||
Protease Inhibitor Cocktail | Sigma-Aldrich | Cat#P8340 |
Gliptins | Sigma-Aldrich | Cat#DPP4 |
Resource availability
Lead contact
Further information and requests for resources and reagents should be directed to and will be fulfilled by the lead contact, Bingyin Shi ( [email protected] ).
Materials availability
This study did not generate new unique reagents.
Experimental model and subject details
Study design
We performed a randomized, open-label, single-center, clinical trial to evaluate the efficacy of weight loss and improvement of metabolic parameters of an LCD, TRE, and their combination, in adults with MetS. Participants were recruited from Xi’an between July 2020 and September 2020, and the trial was conducted from September 2020 to January 2021. This study was conducted with approval from the Institutional Review Board at the First Affiliated Hospital of Xi’an Jiaotong University, Shaanxi, China. This trial was registered as ClinicalTrials.gov, number NCT04475822.
Participants
Participants were recruited via emails, flyers, social media, and website advertisements and were diagnosed with metabolic syndrome. All participants provided written informed consent.
Inclusion criteria
- (1)Diagnosed with metabolic syndrome (i.e., more than 3 abnormal findings out of 5):
- a.Waist circumference ≥90 cm (men) or ≥80 cm (women).
- b.Elevated TG (use of medications for elevated TG is an alternate indicator) ≥ 150 mg/dL (1.7 mmol/L).
- c.Reduced HDL-c (use of medications for reduced HDL-c is an alternate indicator) < 40 mg/dL (1.0 mmol/L) in males <50 mg/dL (1.3 mmol/L) in females.
- d.Elevated blood pressure (use of hypoglycemic medications is an alternate indicator). SBP ≥130 and/or DBP ≥85 mmHg.
- e.Elevated FBG (used of hypoglycemic medications is an alternate indicator) ≥ 100 mg/dL (5.6 mmol/L).
- a.
- (2)Age from 18 to 65 years.
- (3)Stable weight (change ≤10% current body weight) for 3 months prior to the study.
- (4)If participants were on hypoglycemic medications, hypotensive medications, lipid-lowering medications and cardiovascular medications, dose adjustment was not permitted during the 3-month intervention.
Exclusion criteria
- 1)Pregnant or breast-feeding.
- 2)Night shift workers.
- 3)History of major diseases or related diseases, such as inflammatory disease, rheumatologic disease, adrenal disease, malignancy, type 1 diabetes, cirrhosis, chronic kidney disease, acquired immunodeficiency syndrome, eating disorder, uncontrolled psychiatric disorder and major adverse cardiovascular event.
- 4)Current participate in other weight-management program, current on a prescribed diet for special disease or current on any drugs that effect appetite.
- 5)History of weight-loss surgery.
Method details
Randomisation and masking
Participants were randomized in a 1:1:1 ratio to an LCD group, TRE group, or combination group (before all baseline measurements). Block randomization was performed by a computer-generated random number list prepared by an investigator without clinical involvement in this trial. After obtaining the patient’s consent, the research nurse telephoned a clinician who was independent of the recruitment process for allocation consignment.
Procedures
Before the intervention started, all participants were requested to maintain their usual diet and physical activity habits for weight stabilization in 2-week. During the 3-month intervention period, the LCD group was instructed to eat a low-carbohydrate diet (carbohydrates <130 g/day or <26% total energy, according to the ADA definition of 130 g/day as recommended minimum); a suggested food and menu list (Table S7) is provided in the supplemental information. The 8 h TRE group was instructed to consume all calories from 8 AM to 4 PM each day and fast from 4 PM to 8 AM, or to consume all calories from 12 PM to 8 PM each day and fast from 8 PM to 12 PM (16 h fast). During the 8 h meal eating windows, they could eat ad libitum without any restriction on the quantities and types of food, and the fasting guide is provided in the supplemental information. Likewise, the combination group was instructed to eat a LCD in the same 8 h meal eating windows as the TRE group. Furthermore, participants were not requested to calculate their caloric intake in 8 h meal eating window. In 16 h fasting window, participants were recommended to drink plenty of water and zero calorie beverages without artificial sweeteners, such as sparkling water and black tea.
The study was conducted with the internet hospital application (App) of the First Affiliated Hospital of Xi’an Jiaotong University, named “Zhihui Hao Yiyuan”, which was a new approach to provide health services, outpatient service in particular, through the internet technology. Participants could contact clinicians at any time and any place though online communication and received diet guides and questionnaires through the App. All the participants were encouraged to write in a daily dietary log and note the time at which they ate with the use of the App, yet this was not compulsory. Clinicians checked participants’ daily dietary log every day, and provided diet guidance in adjusting schemes for compliance based on participants’ dietary interventions through the App. Raw data in Chinese version is available from the lead contact upon reasonable request. All participants were asked to maintain their usual physical activity throughout the study, which was evaluated by International Physical Activity Questionnaire (IPAQ) before and after 3-month intervention. All participants received our own custom-made sport bracelet, recording daily step counts that was connected with our App, and were encouraged to wear it during the 3-month intervention period.
Compliance with the dietary intervention was assessed for all participants every other week through a Food Frequency Questionnaire (FFQ), including days of adherence, meal eating window, and the amount, type and frequency of food intake (a blank copy of FFQ can be found as Data S1 in supplemental information). Compliance with diet was evaluated by the same dietician every other week. “Daily carbohydrate intake” was estimated by the same dietician, according to a previously defined method providing quantitative information on macronutrient composition of the diet consumed. “Meal eating window” was calculated by participants self-report on 2-week recall by FFQ. “Days of adherence” was assessed by participants self-reporting being compliant with their diet intervention during the 3-month intervention period. “Willingness to continue the diet” was evaluated by asking those who completed the intervention their willingness at the end of 3-month intervention.
Outcomes
The primary outcome of the study was changes in body weight and abdominal fat area. Secondary outcomes were changes in body composition, glycemic control, plasma lipids, UA and blood pressure.
Body weight was assessed every month at the research center with the participants without shoes and in light clothing using a digital scale (OMRON MEDICAL Beijing Co., Ltd. HNH-318) to the nearest 0.1 kg. Height was assessed during the screening visit using a wall-mounted stadiometer (OMRON MEDICAL Beijing Co., Ltd. HNH-318) to the nearest 0.1 cm. Abdominal fat area (VFA and SFA) was measured at baseline and after 3 months using bioelectrical impedance analysis (OMRON MEDICAL Beijing Co., Ltd. DUALSCAN, HDS-2000) to the nearest 1 cm2. This approach has been proved to produce reliable measurements that correlate well with data obtained from computed tomography (CT). Body composition (body fat mass and body muscle mass) was measured at baseline and month 3 using the direct segmental multifrequency bioelectrical impedance analysis method DSM-BIA (InBody H20) to the nearest 0.1 kg.
All blood collection was performed at the physical examination center of the First Affiliated Hospital of Xi’an Jiaotong University, after fasting overnight (i.e., from 20:00 on) at baseline and at month 3, between 7:40 and 9:00 am. Blood was centrifuged for 20 min at 1500 g and 4°C to separate plasma, then stored at −80°C until analysis. HbA1c was measured on an automatic HbA1c analyzer (TOSOH BIOSCIENCE, Inc.; HLC-723G8) to the nearest 0.1%. FBG, UA, total cholesterol, TG, HDL-c, and LDL-c were measured on an automatic biochemistry analyzer (HITACHI, Inc.; LAbOSPECT, 008AS) using standard reagents to the nearest 0.01 mmol/L, 1 μmol/L, 0.01 mmol/L, 0.01 mmol/L, 0.01 mmol/L and 0.01 mmol/L, respectively.
Fasting insulin and C-peptide were measured by immunoassay with fluorescent detection on a Luminex instrument (EMD Millipore Corporation; HMHEMAG-34K) to the nearest 0.1 pg/mL. Insulin resistance and insulin sensitivity were calculated using the homeostasis model assessment method by applying the following formula: [HOMA-IR = fasting insulin (mIU/L) × fasting glucose (mg/dL)/405], [HOMA-IS = 1/HOMA-IR]. QUICKI = 1/[log (fasting insulin level, in microunits per milliliter) + log (fasting glucose level, in milligrams per deciliter)]. Blood pressure was measured in triplicate using a digital automatic blood pressure (Omron HBP-9020, Kyoto, Japan) to the nearest 1 mmHg.
Adverse effects (constipation, dizziness, insomnia, dry mouth and alopecia) were assessed by a telephone interview at baseline and every other week during the 3-month intervention.
Statistical analysis
This study was powered to detect the primary outcome of percentage reduction in body weight. We estimated that the LCD-treated group (A) would lose 5% body weight and that the group treated with combination diet (C) would lose 10% of body weight after 3 months. The proposed reduction in body weight were determined on the basis of preliminary data obtained from dietary intervention studies.,,, We calculated that 78 participants (26 per group) would provide with greater than 80% power to detect a significant difference of 5% in body weight between the A and C groups at a significance level of 0.05 using a 2-tailed independent-samples t test. We estimated that dropout rate was 20%. Therefore, we decided to recruit 165 participants (55 per group) to increase our statistical power because our dropout rate might be higher than expected.
Statistical analyses were performed using R version 4.1.3. A two-tailed p value of less than 0.05 was considered statistically significant. Tests for normality were conducted. All data are presented as the mean ± SEM for normally distributed variables or median (interquartile range, IQR) for abnormally distributed variables. At baseline, differences between treatment arms (LCD, TRE and combination) were tested by one-way ANOVA or Kruskal-Wallis H test. Analyses were conducted in the intention-to-treat population, using a linear mixed model with randomized dietary intervention as a factor to correct for the correlations of repeated measurements on changes in body weight, and handled other missing data by multiple imputations with the use of the Markov chain Monte Carlo method. Change scores are represented by “Δ” in the results text. At month 3, pairwise comparisons of change scores from baseline between the groups (e.g., TRE vs. LCD, TRE vs. Combi, LCD vs. Combi) were evaluated by t test or Mann-Whitney U test. The significant difference between baseline and 3-month follow-up was measured by paired T test or Wilcoxon test in each group. Pearson and Spearman correlations were performed to assess the relationship between abdominal fat area and other metabolic risk factors.
The trial protocol can be found as Data S2 in supplemental information.
Data and code availability
- •All data reported in this paper will be shared by the lead contact upon reasonable request.
- •This paper does not report original code.
- •Any additional information required to reanalyze the data reported in this work paper is available from the lead contact upon reasonable request.
Acknowledgments
This study was supported by grants from National Key R&D Program of China (no. 2018YFC1311501 ). Y.-N.W. is supported by the China “ Thousand Talents Plan ” (Young Talents), Shaanxi province “ Thousand Talents Plan ” (Young Talents), and Foundation of Xi’an Jiaotong University (Plan A). We thank Mrs. Alice Chung for helping in dietary instruction.
Author contributions
M.H. and Y.-N.W. conducted the clinical trial, analyzed the data, and wrote the manuscript; B.S., C.-C.H., and P.C.N.R. designed the research and revised the manuscript; J.W., Q.L., M.L., H.G., Y.W., C.D., J.S., Y.Z., and Y.-W.W. assisted with the conduction of the clinical trial; B.Q., H.C., M.M., S.S., H.G., W.-X.Z., and X.G. conducted the investigation; Y.L., W.-Z.Z., M.Z., and Z.C. assisted with the statistical analysis. All authors helped interpret the data, revised the manuscript for critical content, and approved the final version of the manuscript.
Declaration of interests
The authors declare no competing interests.
Supplemental information
- Document S1. Figures S1, S2, Tables S1–S7, Data S1 and S2
References
- Sperling L.S.
- Mechanick J.I.
- Neeland I.J.
- Herrick C.J.
- Després J.P.
- Ndumele C.E.
- Vijayaraghavan K.
- Handelsman Y.
- Puckrein G.A.
- Araneta M.R.G.
- et al.
The CardioMetabolic health alliance: working toward a new care model for the metabolic syndrome.J. Am. Coll. Cardiol. 2015; 66: 1050-1067- Alberti K.G.M.M.
- Eckel R.H.
- Grundy S.M.
- Zimmet P.Z.
- Cleeman J.I.
- Donato K.A.
- Fruchart J.C.
- James W.P.T.
- Loria C.M.
- Smith Jr., S.C.
- et al.
Harmonizing the metabolic syndrome: a joint interim statement of the international diabetes federation task force on epidemiology and prevention; national heart, lung, and blood institute; American heart association; world heart federation; international atherosclerosis society; and international association for the study of obesity.Circulation. 2009; 120: 1640-1645- Wilson P.W.F.
- D'Agostino R.B.
- Parise H.
- Sullivan L.
- Meigs J.B.
Metabolic syndrome as a precursor of cardiovascular disease and type 2 diabetes mellitus.Circulation. 2005; 112: 3066-3072- Teng D.
- Shi X.
- Qin G.
- Qin Y.
- Quan H.
- Shi B.
- Sun H.
- Chen B.
- et al.
Prevalence of diabetes recorded in mainland China using 2018 diagnostic criteria from the American Diabetes Association: national cross sectional study.BMJ. 2020; 369: m997- Zhao D.
- Liu J.
- Wang M.
- Zhang X.
- Zhou M.
Epidemiology of cardiovascular disease in China: current features and implications.Nat. Rev. Cardiol. 2019; 16: 203-212- Heymsfield S.B.
- Wadden T.A.
Mechanisms, pathophysiology, and management of obesity.N. Engl. J. Med. 2017; 376: 254-266- Brown J.D.
- Buscemi J.
- Milsom V.
- Malcolm R.
- O'Neil P.M.
Effects on cardiovascular risk factors of weight losses limited to 5-10.Transl. Behav. Med. 2016; 6: 339-346- Yancy Jr., W.S.
- Olsen M.K.
- Guyton J.R.
- Bakst R.P.
- Westman E.C.
A low-carbohydrate, ketogenic diet versus a low-fat diet to treat obesity and hyperlipidemia: a randomized, controlled trial.Ann. Intern. Med. 2004; 140: 769-777- Samaha F.F.
- Iqbal N.
- Seshadri P.
- Chicano K.L.
- Daily D.A.
- McGrory J.
- Williams T.
- Williams M.
- Gracely E.J.
- Stern L.
A low-carbohydrate as compared with a low-fat diet in severe obesity.N. Engl. J. Med. 2003; 348: 2074-2081- Gower B.A.
- Goss A.M.
A lower-carbohydrate, higher-fat diet reduces abdominal and intermuscular fat and increases insulin sensitivity in adults at risk of type 2 diabetes.J. Nutr. 2015; 145: 177s-183s- Goss A.M.
- Goree L.L.
- Ellis A.C.
- Chandler-Laney P.C.
- Casazza K.
- Lockhart M.E.
- Gower B.A.
Effects of diet macronutrient composition on body composition and fat distribution during weight maintenance and weight loss.Obesity. 2013; 21: 1139-1142- Buyken A.E.
- Mela D.J.
- Dussort P.
- Johnson I.T.
- Macdonald I.A.
- Stowell J.D.
- Brouns F.J.P.H.
Dietary carbohydrates: a review of international recommendations and the methods used to derive them.Eur. J. Clin. Nutr. 2018; 72: 1625-1643- Wylie-Rosett J.
- Apovian C.M.
- Franz M.J.
- Lichtenstein A.H.
- Mooradian A.D.
- Hoogwerf B.J.
- Lichtenstein A.H.
- Mayer-Davis E.
- Mooradian A.D.
- Wheeler M.L.
- American Diabetes Association
Nutrition recommendations and interventions for diabetes: a position statement of the American Diabetes Association.Diabetes Care. 2008; 31: S61-S78- Feinman R.D.
- Pogozelski W.K.
- Astrup A.
- Bernstein R.K.
- Fine E.J.
- Westman E.C.
- Accurso A.
- Frassetto L.
- Gower B.A.
- McFarlane S.I.
- et al.
Dietary carbohydrate restriction as the first approach in diabetes management: critical review and evidence base.Nutrition. 2015; 31: 1-13- Hjorth M.F.
- Ritz C.
- Blaak E.E.
- Saris W.H.
- Langin D.
- Poulsen S.K.
- Larsen T.M.
- Sørensen T.I.
- Zohar Y.
- Astrup A.
Pretreatment fasting plasma glucose and insulin modify dietary weight loss success: results from 3 randomized clinical trials.Am. J. Clin. Nutr. 2017; 106: 499-505- Wilkinson M.J.
- Manoogian E.N.C.
- Zadourian A.
- Fakhouri S.
- Shoghi A.
- Wang X.
- Fleischer J.G.
- Navlakha S.
- Panda S.
- Taub P.R.
Ten-hour time-restricted eating reduces weight, blood pressure, and atherogenic lipids in patients with metabolic syndrome.Cell Metabol. 2020; 31: 92-104.e5- Cienfuegos S.
- Gabel K.
- Kalam F.
- Ezpeleta M.
- Wiseman E.
- Pavlou V.
- Lin S.
- Oliveira M.L.
- Varady K.A.
Effects of 4- and 6-h time-restricted feeding on weight and cardiometabolic health: a randomized controlled trial in adults with obesity.Cell Metabol. 2020; 32: 366-378.e3- Hutchison A.T.
- Regmi P.
- Manoogian E.N.C.
- Fleischer J.G.
- Wittert G.A.
- Panda S.
- Heilbronn L.K.
Time-restricted feeding improves glucose tolerance in men at risk for type 2 diabetes: a randomized crossover trial.Obesity. 2019; 27: 724-732- Sutton E.F.
- Beyl R.
- Early K.S.
- Cefalu W.T.
- Ravussin E.
- Peterson C.M.
Early time-restricted feeding improves insulin sensitivity, blood pressure, and oxidative stress even without weight loss in men with prediabetes.Cell Metabol. 2018; 27: 1212-1221.e3- de Cabo R.
- Mattson M.P.
Effects of intermittent fasting on health, aging, and disease.N. Engl. J. Med. 2019; 381: 2541-2551- Patterson R.E.
- Sears D.D.
Metabolic effects of intermittent fasting.Annu. Rev. Nutr. 2017; 37: 371-393- Lunn R.M.
- Blask D.E.
- Coogan A.N.
- Figueiro M.G.
- Gorman M.R.
- Hall J.E.
- Hansen J.
- Nelson R.J.
- Panda S.
- Smolensky M.H.
- et al.
Health consequences of electric lighting practices in the modern world: a report on the National Toxicology Program's workshop on shift work at night, artificial light at night, and circadian disruption.Sci. Total Environ. 2017; 607-608: 1073-1084- Mohebbi I.
- Shateri K.
- Seyedmohammadzad M.
The relationship between working schedule patterns and the markers of the metabolic syndrome: comparison of shift workers with day workers.Int. J. Occup. Med. Environ. Health. 2012; 25: 383-391- Puttonen S.
- Härmä M.
- Hublin C.
Shift work and cardiovascular disease - pathways from circadian stress to morbidity.Scand. J. Work. Environ. Health. 2010; 36: 96-108- Panda S.
Circadian physiology of metabolism.Science. 2016; 354: 1008-1015- Longo V.D.
- Panda S.
Fasting, circadian rhythms, and time-restricted feeding in healthy lifespan.Cell Metabol. 2016; 23: 1048-1059- Hou T.
- Duncan M.J.
- Olga V.A.
- Guo Z.
- Gong M.C.
Time-restricted feeding protects the blood pressure circadian rhythm in diabetic mice.Proc. Natl. Acad. Sci. USA. 2021; 118- Ulgherait M.
- Midoun A.M.
- Park S.J.
- Gatto J.A.
- Tener S.J.
- Siewert J.
- Klickstein N.
- Canman J.C.
- Ja W.W.
- Shirasu-Hiza M.
Circadian autophagy drives iTRF-mediated longevity.Nature. 2021; 598: 353-358- Sato J.
- Kanazawa A.
- Makita S.
- Hatae C.
- Komiya K.
- Shimizu T.
- Ikeda F.
- Tamura Y.
- Ogihara T.
- Mita T.
- et al.
A randomized controlled trial of 130 g/day low-carbohydrate diet in type 2 diabetes with poor glycemic control.Clin. Nutr. 2017; 36: 992-1000- Zhang X.
- Zhang M.
- Zhao Z.
- Huang Z.
- Deng Q.
- Pan A.
- Chen Z.
- Zhou M.
- et al.
Geographic variation in prevalence of adult obesity in China: results from the 2013-2014 national chronic disease and risk factor surveillance.Ann. Intern. Med. 2020; 172: 291-293- Yusuf S.
- Hawken S.
- Ounpuu S.
- Bautista L.
- Franzosi M.G.
- Commerford P.
- Lang C.C.
- Rumboldt Z.
- Onen C.L.
- Lisheng L.
- et al.
Obesity and the risk of myocardial infarction in 27, 000 participants from 52 countries: a case-control study.Lancet (London, England). 2005; 366: 1640-1649- See R.
- Abdullah S.M.
- McGuire D.K.
- Khera A.
- Patel M.J.
- Lindsey J.B.
- Grundy S.M.
- de Lemos J.A.
The association of differing measures of overweight and obesity with prevalent atherosclerosis: the Dallas Heart Study.J. Am. Coll. Cardiol. 2007; 50: 752-759- Schmidt S.
- Christensen M.B.
- Serifovski N.
- Damm-Frydenberg C.
- Jensen J.E.B.
- Fløyel T.
- Størling J.
- Ranjan A.
- Nørgaard K.
Low versus high carbohydrate diet in type 1 diabetes: a 12-week randomized open-label crossover study.Diabetes Obes. Metabol. 2019; 21: 1680-1688- Abbasi J.
Interest in the ketogenic diet grows for weight loss and type 2 diabetes.JAMA. 2018; 319: 215-217- Foster G.D.
- Wyatt H.R.
- Hill J.O.
- McGuckin B.G.
- Brill C.
- Mohammed B.S.
- Szapary P.O.
- Rader D.J.
- Edman J.S.
- Klein S.
A randomized trial of a low-carbohydrate diet for obesity.N. Engl. J. Med. 2003; 348: 2082-2090- Bazzano L.A.
- Reynolds K.
- Yao L.
- Bunol C.
- Liu Y.
- Chen C.S.
- Klag M.J.
- Whelton P.K.
Effects of low-carbohydrate and low-fat diets: a randomized trial.Ann. Intern. Med. 2014; 161: 309-318- Goldenberg J.Z.
- Day A.
- Brinkworth G.D.
- Sato J.
- Yamada S.
- Jönsson T.
- Beardsley J.
- Johnson J.A.
- Thabane L.
- Johnston B.C.
Efficacy and safety of low and very low carbohydrate diets for type 2 diabetes remission: systematic review and meta-analysis of published and unpublished randomized trial data.BMJ. 2021; 372: m4743- Liu D.
- Huang Y.
- Huang C.
- Yang S.
- Wei X.
- Zhang P.
- Guo D.
- Lin J.
- et al.
Calorie restriction with or without time-restricted eating in weight loss.N. Engl. J. Med. 2022; 386: 1495-1504- Gabel K.
- Hoddy K.K.
- Haggerty N.
- Song J.
- Kroeger C.M.
- Trepanowski J.F.
- Panda S.
- Varady K.A.
Effects of 8-hour time restricted feeding on body weight and metabolic disease risk factors in obese adults: a pilot study.Nutr. Healthy Aging. 2018; 4: 345-353- Gill S.
- Panda S.
A smartphone App reveals erratic diurnal eating patterns in humans that can Be modulated for health benefits.Cell Metabol. 2015; 22: 789-798- Williamson D.A.
- Bray G.A.
- Ryan D.H.
Is 5% weight loss a satisfactory criterion to define clinically significant weight loss?.Obesity. 2015; 23: 2319-2320- Pou K.M.
- Massaro J.M.
- Hoffmann U.
- Vasan R.S.
- Maurovich-Horvat P.
- Larson M.G.
- Keaney Jr., J.F.
- Meigs J.B.
- Lipinska I.
- Kathiresan S.
- et al.
Visceral and subcutaneous adipose tissue volumes are cross-sectionally related to markers of inflammation and oxidative stress: the Framingham Heart Study.Circulation. 2007; 116: 1234-1241- Hajer G.R.
- van Haeften T.W.
- Visseren F.L.J.
Adipose tissue dysfunction in obesity, diabetes, and vascular diseases.Eur. Heart J. 2008; 29: 2959-2971- Tchernof A.
- Després J.P.
Pathophysiology of human visceral obesity: an update.Physiol. Rev. 2013; 93: 359-404- Hayes M.R.
- Miller C.K.
- Ulbrecht J.S.
- Mauger J.L.
- Parker-Klees L.
- Gutschall M.D.
- Mitchell D.C.
- Smiciklas-Wright H.
- Covasa M.
A carbohydrate-restricted diet alters gut peptides and adiposity signals in men and women with metabolic syndrome.J. Nutr. 2007; 137: 1944-1950- Di Bonito P.
- Valerio G.
- Licenziati M.R.
- Campana G.
- Del Giudice E.M.
- Di Sessa A.
- Morandi A.
- Maffeis C.
- Chiesa C.
- Pacifico L.
- et al.
Uric acid, impaired fasting glucose and impaired glucose tolerance in youth with overweight and obesity.Nutr. Metabol. Cardiovasc. Dis. 2021; 31: 675-680- Yu T.Y.
- Jee J.H.
- Bae J.C.
- Jin S.M.
- Baek J.H.
- Lee M.K.
- Kim J.H.
Serum uric acid: a strong and independent predictor of metabolic syndrome after adjusting for body composition.Metabolism. 2016; 65: 432-440- Cheng D.
- Lin L.
- Peng K.
- et al.
Serum uric acid and risk of incident diabetes in middle-aged and elderly Chinese adults: prospective cohort study.Front. Med. 2020; 14: 802-810- Mattson M.P.
- Moehl K.
- Ghena N.
- Schmaedick M.
- Cheng A.
Intermittent metabolic switching, neuroplasticity and brain health.Nat. Rev. Neurosci. 2018; 19: 63-80- Huet C.
- Boudaba N.
- Guigas B.
- Viollet B.
- Foretz M.
Glucose availability but not changes in pancreatic hormones sensitizes hepatic AMPK activity during nutritional transition in rodents.J. Biol. Chem. 2020; 295: 5836-5849- Bowen J.
- Brindal E.
- James-Martin G.
- Noakes M.
Randomized trial of a high protein, partial meal replacement program with or without alternate day fasting: similar effects on weight loss, retention status, nutritional, metabolic, and behavioral outcomes.Nutrients. 2018; 10: E1145- Moro T.
- Tinsley G.
- Bianco A.
- Marcolin G.
- Pacelli Q.F.
- Battaglia G.
- Palma A.
- Gentil P.
- Neri M.
- Paoli A.
Effects of eight weeks of time-restricted feeding (16/8) on basal metabolism, maximal strength, body composition, inflammation, and cardiovascular risk factors in resistance-trained males.J. Transl. Med. 2016; 14: 290- Harvie M.N.
- Pegington M.
- Mattson M.P.
- Frystyk J.
- Dillon B.
- Evans G.
- Cuzick J.
- Jebb S.A.
- Martin B.
- Cutler R.G.
- et al.
The effects of intermittent or continuous energy restriction on weight loss and metabolic disease risk markers: a randomized trial in young overweight women.Int. J. Obes. 2011; 35: 714-727- Wood R.J.
- Volek J.S.
- Liu Y.
- Shachter N.S.
- Contois J.H.
- Fernandez M.L.
Carbohydrate restriction alters lipoprotein metabolism by modifying VLDL, LDL, and HDL subfraction distribution and size in overweight men.J. Nutr. 2006; 136: 384-389- Volek J.S.
- Fernandez M.L.
- Feinman R.D.
- Phinney S.D.
Dietary carbohydrate restriction induces a unique metabolic state positively affecting atherogenic dyslipidemia, fatty acid partitioning, and metabolic syndrome.Prog. Lipid Res. 2008; 47: 307-318- Westman E.C.
- Yancy Jr., W.S.
- Olsen M.K.
- Dudley T.
- Guyton J.R.
Effect of a low-carbohydrate, ketogenic diet program compared to a low-fat diet on fasting lipoprotein subclasses.Int. J. Cardiol. 2006; 110: 212-216- Bhanpuri N.H.
- Hallberg S.J.
- Williams P.T.
- McKenzie A.L.
- Ballard K.D.
- Campbell W.W.
- McCarter J.P.
- Phinney S.D.
- Volek J.S.
Cardiovascular disease risk factor responses to a type 2 diabetes care model including nutritional ketosis induced by sustained carbohydrate restriction at 1 year: an open label, non-randomized, controlled study.Cardiovasc. Diabetol. 2018; 17: 56- Trepanowski J.F.
- Kroeger C.M.
- Barnosky A.
- Klempel M.C.
- Bhutani S.
- Hoddy K.K.
- Gabel K.
- Freels S.
- Rigdon J.
- Rood J.
- et al.
Effect of alternate-day fasting on weight loss, weight maintenance, and cardioprotection among metabolically healthy obese adults: a randomized clinical trial.JAMA Intern. Med. 2017; 177: 930-938- Wang Y.
- Snel M.
- Jonker J.T.
- Hammer S.
- Lamb H.J.
- de Roos A.
- Meinders A.E.
- Pijl H.
- Romijn J.A.
- Smit J.W.A.
- et al.
Prolonged caloric restriction in obese patients with type 2 diabetes mellitus decreases plasma CETP and increases apolipoprotein AI levels without improving the cholesterol efflux properties of HDL.Diabetes Care. 2011; 34: 2576-2580- Kim J.Y.
- Kim S.M.
- Kim S.J.
- Lee E.Y.
- Kim J.R.
- Cho K.H.
Consumption of policosanol enhances HDL functionality via CETP inhibition and reduces blood pressure and visceral fat in young and middle-aged subjects.Int. J. Mol. Med. 2017; 39: 889-899- Zomer E.
- Gurusamy K.
- Leach R.
- Trimmer C.
- Lobstein T.
- Morris S.
- James W.P.T.
- Finer N.
Interventions that cause weight loss and the impact on cardiovascular risk factors: a systematic review and meta-analysis.Obes. Rev. 2016; 17: 1001-1011- Lowe D.A.
- Rohdin-Bibby L.
- Moore A.H.
- Kelly N.
- Liu Y.E.
- Philip E.
- Vittinghoff E.
- Heymsfield S.B.
- Olgin J.E.
- et al.
Effects of time-restricted eating on weight loss and other metabolic parameters in women and men with overweight and obesity: the TREAT randomized clinical trial.JAMA Intern. Med. 2020; 180: 1491-1499- Xie Z.
- Sun Y.
- Zhang H.
- Zhao H.
- Yang H.
- Mao Y.
Randomized controlled trial for time-restricted eating in healthy volunteers without obesity.Nat. Commun. 2022; 13: 1003- Morris C.J.
- Garcia J.I.
- Myers S.
- Yang J.N.
- Trienekens N.
- Scheer F.A.J.L.
The human circadian system has a dominating role in causing the morning/evening difference in diet-induced thermogenesis.Obesity. 2015; 23: 2053-2058- Poggiogalle E.
- Jamshed H.
- Peterson C.M.
Circadian regulation of glucose, lipid, and energy metabolism in humans.Metabolism. 2018; 84: 11-27- Scheer F.A.J.L.
- Hilton M.F.
- Mantzoros C.S.
- Shea S.A.
Adverse metabolic and cardiovascular consequences of circadian misalignment.Proc. Natl. Acad. Sci. USA. 2009; 106: 4453-4458- Morris C.J.
- Yang J.N.
- Garcia J.I.
- Myers S.
- Bozzi I.
- Wang W.
- Buxton O.M.
- Shea S.A.
- Scheer F.A.J.L.
Endogenous circadian system and circadian misalignment impact glucose tolerance via separate mechanisms in humans.Proc. Natl. Acad. Sci. USA. 2015; 112: E2225-E2234- Ravussin E.
- Beyl R.A.
- Poggiogalle E.
- Hsia D.S.
- Peterson C.M.
Early time-restricted feeding reduces appetite and increases fat oxidation but does not affect energy expenditure in humans.Obesity. 2019; 27: 1244-1254- Ida M.
- Hirata M.
- Odori S.
- Mori E.
- Kondo E.
- Fujikura J.
- Kusakabe T.
- Ebihara K.
- Hosoda K.
- Nakao K.
Early changes of abdominal adiposity detected with weekly dual bioelectrical impedance analysis during calorie restriction.Obesity. 2013; 21: E350-E353- Katz A.
- Nambi S.S.
- Mather K.
- Baron A.D.
- Follmann D.A.
- Sullivan G.
- Quon M.J.
Quantitative insulin sensitivity check index: a simple, accurate method for assessing insulin sensitivity in humans.J. Clin. Endocrinol. Metab. 2000; 85: 2402-2410- Shai I.
- Schwarzfuchs D.
- Henkin Y.
- Shahar D.R.
- Witkow S.
- Greenberg I.
- Golan R.
- Fraser D.
- Bolotin A.
- Vardi H.
- et al.
Weight loss with a low-carbohydrate, Mediterranean, or low-fat diet.N. Engl. J. Med. 2008; 359: 229-241- Gardner C.D.
- Trepanowski J.F.
- Del Gobbo L.C.
- Hauser M.E.
- Rigdon J.
- Ioannidis J.P.A.
- Desai M.
- King A.C.
Effect of low-fat vs low-carbohydrate diet on 12-month weight loss in overweight Adults and the association with genotype pattern or insulin secretion: the DIETFITS randomized clinical trial.JAMA. 2018; 319: 667-679
Article Info
Publication History
Published: October 18, 2022
Accepted:
September 20,
2022
Received in revised form:
July 24,
2022
Received:
January 2,
2022
Publication stage
In Press, Corrected ProofIdentification
DOI: https://doi.org/10.1016/j.xcrm.2022.100777
Copyright
© 2022 The Authors.
ScienceDirect
Access this article on ScienceDirectFigures
- Graphical Abstract
- Figure 1Trial profile
- Figure 2Body weight and body composition change
- Figure 3Change in metabolic factors among three groups
Related Articles
Recommend
About Joyk
Aggregate valuable and interesting links.
Joyk means Joy of geeK