
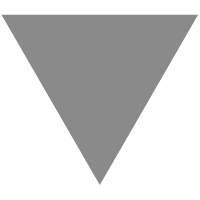
GCC's Assembler Syntax
source link: https://www.tuicool.com/articles/ziEnmyr
Go to the source link to view the article. You can view the picture content, updated content and better typesetting reading experience. If the link is broken, please click the button below to view the snapshot at that time.
GCC's assembler syntax
This page is meant to consolidate GCC's official extended asm syntax into a form that is consumable by mere mortals. It is intended to be accessible by people who know a little bit of C and a little bit of assembly.
Guide to this guide
This documentation is better viewed on a desktop computer.
Right below is the index of this page. Beyond that, here is some useful notation:
-
Non-trivial
asm
examples come with a [ godbolt ] link. Matt Godbolt's website hosts Compiler Explorer , a very useful resource to check out how compilers handle some given input. - Instructions link to the x86 instruction documentation that is also hosted on this website.
- asm arguments are co lo red to be more easily identifiable.
- Constraint strings have their background highlighted and have a tooltip that explains the constraint.
Index
Syntax overview
asm
is a statement
, not an expression.
asm <optional stuff> ( "assembler template" : outputs : inputs : clobbers : labels)
Quick notes:
-
the starting
asm
keyword is eitherasm
or__asm__
, at your convenience -
<optional stuff>
may be empty, or the keyword(s)volatile
orgoto
(explained below) -
"assembler template"
is a required string that encodes the instruction(s) that you want to run (explained below) -
there is a combined maximum of 30 inputs and outputs per
asm
statement - outputs, inputs, clobbers, and labels are all optional. Colons are only required up to the parameter that you wish to use, and having nothing between colons is valid when you wish to skip a parameter. For instance, each of these (explained below) are valid:
Assembler template syntax
The assembler template contains instructions and operand placeholders. You should think of it as a format string. This string will be "printed" to the assembly file that the compiler generates. Instructions must be separated by a newline (literally \n
), and it's popular to prefix them with a tab or spaces (although this is not necessary). The template is a good use case for C's string literal concatenation
:
asm("<a href="/x86/NOP.html">nop</a>\n" "<a href="/x86/NOP.html">nop</a>\n" "<a href="/x86/NOP.html">nop</a>\n")
In this format string:
-
you specify arguments using
%N
, whereN
refers to an argument by its zero-based number in order of appearance (outputs and inputs being in the same "namespace", outputs being first) -
you specify named arguments using
%[Name]
(see below for how to specify names) -
you specify a literal % by using
%%
, which you will need if you reference registers directly in x86 assembly with the AT&T syntax -
the GCC documentation additionally specifies
%=
,{=
,%|
and%}
, which this page does not cover
Outputs and inputs
Outputs and inputs are the parameters to your assembler template "format string". They are comma-separated. They both use either one of the two following patterns:
"constraint" (expression) [Name] "constraint" (expression)
Note that although this looks metasyntactic, these are in fact the literal spellings that you must use: [Name]
(when used) needs
to be enclosed in square brackets, "constraint"
needs
to be enclosed in double quotes, and (expression)
needs
to be enclosed in parentheses.
"constraints"
, and the kind of (expression)
s that are valid, are explained in the,andsections below.
When you specify the optional [Name]
field, you become able to refer to that input or output using the %[Name]
syntax in the assembler template. For instance:
int foo = 1; asm("inc %[IncrementMe]" : [IncrementMe] "+r" (foo)); // foo == 2
Even when names are specified, you can still refer to operands using the numbered syntax. Corollary: named arguments contribute to the sequence of numbered arguments. The second argument of an asm
statement is always available as %1
, regardless of whether the first argument has a name or not.
Constraints
Constraint strings bridge your C expressions to assembler operands. They are necessary because the compiler doesn't know what kind of operands are valid for what instructions. In fact, operands aren't even required to be instruction operands: you could use them in comments or instruction names, as long as the output is valid to the assembler, for all the compiler cares.
For a valid example, in "imul %0, %1, %2"
:
- the first operand has to be a register
- the second operand may be a register or a memory address
- the last operand has to be a constant integer value
The constraint string for each operand must communicate these requirements to GCC. For instance, it will ensure that the destination value lives in a register that can be used at the point of this statement.
GCC defines many types of constraints, but on 2019's desktop/mobile platforms, those are the constraints that are the most likely to be used:
- r specifies that the operand must be a general-purpose register
- m specifies that the operand must be a memory address
- i specifies that the operand must be an integer constant
- g specifies that the operand must be a general-purpose register, or a memory address, or an integer constant (effectively the same as "rmi" )
As implied by the g
constraint, it is possible to specify multiple constraints for each argument. By specifying multiple constraints, you allow the compiler to pick the operand kind that suits it best when the same instruction has multiple forms. This is useful on x86 and not so much on ARM, because x86 overloads mnemonics with many operand types.
For example, in this code:
int add(int a, int b) { asm("addl %1, %0" : "+r" (a) : "rm" (b)); return a; }
This is how the compiler could choose to satisfy the r
constraint (although, to be clear, it may well use a less efficient way):
add: // Per x86_64 System V calling convention: // * a is held in edi. // * b is held in esi. // The return value will be held in eax. // The compiler chooses to move `a` to eax before // the add (it could arbitrarily do it after). <a href="/x86/MOV.html">movl</a> %edi, %eax // `b` does not need to be moved anywhere, it is // already in a register. // The compiler can emit the addition. <a href="/x86/ADD.html">addl</a> %esi, %eax // The result of the addition is returned. <a href="/x86/RET.html">ret</a>
Note that when it comes to i
, the satisfiability of the constraint may depend on your optimization levels. Passing an integer literal or an enum value always works, but when it comes to variables, it depends on the compiler's ability to fold constants. For instance, this will work at -O1
and above, but not -O0
, because the compiler needs to establish that x
has a constant value:
int x = 3; asm volatile("int %0" :: "i" (x) : "memory"); // [godbolt] produces "int 3" at -O1 and above; // [godbolt] errors out at -O0
GCC documents the full list of platform-independent constraints , as well as the full list of platform-specific constraints .
Outputs
Outputs specify lvalues
where the result should be stored at the end of the operation. As a refresher, the concept of lvalue in C is nebulous, but something that is assignable is usually an lvalue (variables, dereferenced pointers, array subscripts, structure fields, etc). Most lvalues are accepted as operands, as long as the constraint can be respected: for instance, it's possible to use a bitfield with r
(register), but not with m
(memory) as you cannot take the address of a bitfield. (The same applies to Clang's vector types
).
In addition, the constraint string of an output must
be prefixed with either =
or +
.
= +
For instance, almost all x86 instructions read and write to their first operand: you need to use the +
prefix to communicate that. A simple example would be:
asm("addl %1, %0" : "+rm" (foo) : "g" (bar))
The initial value of foo
is important here, because it's what bar
will be added to. (The whole constraint string additionally specifies that foo
may be referenced in the assembler string as a register or a memory address, since x86 has instruction forms for both.)
On the other hand, almost no ARM instruction uses the initial value of its destination register. In those cases, you would use =
to communicate that. A simple example would be:
asm("add %0, %1, %2" : "=r" (foo) : "r" (bar), "r" (baz))
(ARM only supports computations on registers, so all the operands are register operands.)
You may additionally request the result of a condition code through an output. The constraint format must be =@ccCOND
, where COND
is the architecture-dependent name of the condition that you wish to test for. For instance, on x86, =@ccnz
will fill your output with, essentially, the result of thesetnz instruction (true if the result is non-zero, false otherwise).
Inputs
Inputs can be any value, as long as it makes sense for the constraint. For instance, to use the i
constraint, the compiler must be able to find that the value is a constant. They are not prefixed with anything.
The use of volatile
IMPORTANT!
-
If the compiler determines that the inputs of your
asm
statement never change, it may move the asm statement (out of a loop, for instance). -
If the compiler determines that the outputs of your
asm
statement are not used, it may REMOVE the asm statement .
If you intend to prevent this, you should either make sure that each output is properly communicated. If you modify some
memory which is not directly related to an output, you may use the "memory"
clobber parameter, as.
Finally, as the biggest hammer in your toolbox, you may add the volatile
modifier to your asm
statement to prevent it from being moved around or removed.
asm volatile("<a href="/x86/MFENCE.html">mfence</a>");
Clobbers
Clobbers are the list of writable locations that the assembly code might have modified, and which have not been specified in outputs. These can be:
-
Register names (on x86, both
register
and%register
are accepted, such asrax
or%rax
) -
The special name
cc
, which specifies that the assembly altered condition flags. On platforms that keep multiple sets of condition flags as separate registers, it's also possible to name indvidual registers: for instance, on PowerPC, you can specify that you clobbercr0
. -
The special name
memory
, which specifies that the assembly wrote to memory that is not directly visible to the compiler (for instance, by dereferencing a pointer input). Amemory
clobber forces the compiler to be pessimistic about memory operations.
The compiler will generate a diagnostic if you attempt to use an unknown clobber location.
Some architectures implicitly clobber some registers on any asm
statement, with no way of opting out. One relevant example is that on x86, the flags register is always clobbered. (A previous version of this document recommended that all asm
statements for x86 clobber cc
.) The compiler will not emit a diagnostic if you explicitly clobber a register that is also implicitly clobbered. There does not appear to be a documented list of per-architecture adjustments to clobbers: the source informs that at the time of writing (October 2019), 7 architectures do it (CRIS, x86, MN103, NDS32, PDP11, IBM RS/6000, Visium), but this author is not familiar enough with most of them to tell what's going on.
As an example of clobbering, theimul instruction takes a single operand but modifies rax
and rdx
implicitly. You would have to specify them in the clobber list to ensure that the compiler doesn't keep anything it needs later in either of those registers.
// [godbolt] asm( "movq %[left], %%rax\n" "imulq %[right]\n" "movq %%rax, %[low]\n" "movq %%rdx, %[high]\n" : [low] "=rm" (low), [high] "=rm" (high) : [left] "g" (left), [right] "rm" (right) : "rax", "rdx")
Labels and the goto
keyword
It's always possible to branch within your asm
code. With a little bit of extra effort, it's also possible to branch out
of your asm
statement, to labels that are available to your enclosing C function. To achieve this, you use asm goto
.
When you use asm goto
, it becomes impossible to specify outputs (this quirk is due to fairly fundamental decisions in the internal code representation of GCC), and you become able to specify labels as a fourth kind of parameter in your asm
statement. Label arguments do not have constraints and cannot be named: they must be referred to by %N
. For example:
// [godbolt] int add_overflows(long lhs, long rhs) { asm goto( "movq %%rax, %[left]\n " "addq %[right], %%rax\n " "jo %2" : // can't have outputs : [left] "g" (lhs), [right] "g" (rhs) : "rax" : on_overflow); return 0; // no overflow on_overflow: return 1; // had an overflow }
(This example's functionality can arguably be replicated with a flag output operand . Seeabove.)
Labels can only be C labels: they can't be other code addresses, such as functions or an indirect goto label variable.
Note that an asm goto
statement is always implicitly volatile
.
Fancy examples
All examples are x86_64. Most x86 instructions clobber CPU flags, so almost all examples here have the cc
clobber.
This section additionally uses x86-specific constraints that bind to named registers:
-
a
, b
, c
, d
: the
a
,b
,c
,d
registers, respectively (the "a
" register being contextuallyal
,ax
,eax
orrax
, for example) -
D
: the
di
register -
S
: the
si
register
Rotate left
This example left-rotates a 64-bit integer. The encoding of rol
is somewhat tricky, allowing an immediate for the count, or (very specifically) the cl
register.
[godbolt] int rotate_left(unsigned long long value, unsigned char count) { asm("rolq %[count], %0" : "+a" (value) : [count] "ci" (count)); return value; }
Do a two-word multiplication
Multiply two 64-bit integers and return the 128-bit result (by address). Here we use the x86-specific a
and d
constraints, which specify "the rax
register" and "the rdx
register", respectively. The variant of the register is chosen by the compiler to be size-appropriate; 32-bit values get eax
, for instance.
This example is creative because it has implicitly-referenced outputs. Using the =d
output constraint to bind *hi
, we cause the compiler to move the value of rdx
to *hi
at the end of the assembly code.
// [godbolt] void imul128( int64_t left, int64_t right, int64_t* lo, int64_t* hi) { asm( "movq %[lhs], %0\n " "imulq %[rhs]" : "=a" (*lo), "=d" (*hi) : [lhs] "g" (left), [rhs] "rm" (right)); }
Call the Linux write
system call
By binding inputs to registers using x86-specific constraints, we let the compiler do any necessary lifting to move argument values to the syscall argument registers. On x86_64 Linux, in the normal case, no moving at all is required for the first 4 arguments.
// [godbolt] int do_write(int fp, void* ptr, size_t size) { int retval; asm volatile( "movl %[read], %%eax\n " "syscall" : "=a"(retval) : "D" (fp), "S" (ptr), "d" (size), [read] "i" (SYS_write) : "rcx", "r11"); return retval; }
Recommend
About Joyk
Aggregate valuable and interesting links.
Joyk means Joy of geeK