
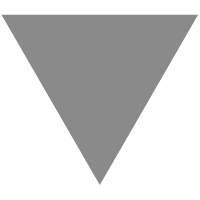
Ion Thrusters: Not Just For TIE Fighters Anymore
source link: https://hackaday.com/2022/03/03/ion-thrusters-not-just-for-tie-fighters-anymore/
Go to the source link to view the article. You can view the picture content, updated content and better typesetting reading experience. If the link is broken, please click the button below to view the snapshot at that time.
Ion Thrusters: Not Just For TIE Fighters Anymore
Spacecraft rocket engines come in a variety of forms and use a variety of fuels, but most rely on chemical reactions to blast propellants out of a nozzle, with the reaction force driving the spacecraft in the opposite direction. These rockets offer high thrust, but they are relatively fuel inefficient and thus, if you want a large change in velocity, you need to carry a lot of heavy fuel. Getting that fuel into orbit is costly, too!
Ion thrusters, in their various forms, offer an alternative solution – miniscule thrust, but high fuel efficiency. This tiny push won’t get you off the ground on Earth. However, when applied over a great deal of time in the vacuum of space, it can lead to a huge change in velocity, or delta V.
This manner of operation means that an ion thruster and a small mass of fuel can theoretically create a much larger delta-V than chemical rockets, perfect for long-range space missions to Mars and other applications, too. Let’s take a look at how ion thrusters work, and some of their interesting applications in the world of spacecraft!
It’s All About Specific Impulse
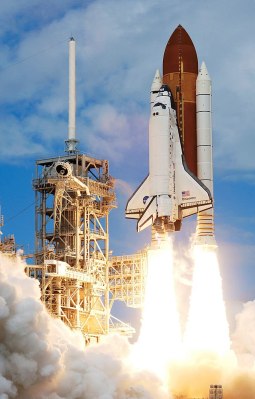
Ion engines won’t get you out of Earth’s gravity well, nor do they work in the atmosphere, but become useful when you’re in the vacuum of space. Credit: NASA, public domain
Before we dive into the world of ion thrusters, it’s important to understand the concept of specific impulse and fuel efficiency for rocket thrusters of all kinds. Specific impulse measures how effectively a rocket engine creates thrust from the mass it throws out the back, whether by chemical or any other means. The higher the specific impulse of a rocket thruster, the more thrust it generates per mass of fuel.
Impulse is the integral of force over time, measured in Newton-seconds. Specific impulse, where we look at impulse per weight of propellant, is thus measured in Newton-seconds divided by Newtons, or simply seconds. It’s a little confusing to wrap your head around, but for the newly initiated, just keep in mind that higher numbers of specific impulse stand for greater fuel efficiency.
For comparison’s sake, the Space Shuttle’s Solid Rocket Boosters get a specific impulse of just 250 seconds, while liquid oxygen-liquid hydrogen rocket engines may reach closer to 450 seconds. Electrostatic ion thrusters are almost an order of magnitude better, on the order of 2,000-3,000 seconds, with some reaching closer to 10,000 seconds in experiments, while the experimental VASIMR electromagnetic ion thruster predicts a specific impulse up to 12,000 seconds.
This better fuel efficiency has real implications for space travel. It means that an ion thruster can achieve a given change in velocity for a space craft with far less fuel – an order of magnitude less, in some regards. In an application regarding orbit-keeping for the ISS, one calculation suggested an ion thruster could reduce the space station’s annual fuel use from 7,500 kg to just 300 kg. This has flow on effects, where launch vehicles carrying that fuel to the space station need less fuel themselves to boost it into orbit, improving efficiency across the board.
How Thrust Via Electricity Works
Ion thrusters come in a variety of forms, but the basic principle is a simple one: electricity is used to accelerate ions to a high velocity, forcing them out of the thruster, thus resulting in a reaction force which propels the spacecraft itself. A neutral gas is used as fuel, which is ionized by stripping electrons from the atoms, resulting in a supply of positive ions that can readily be accelerated by electrostatic or electromagnetic means to generate thrust. Xenon, krypton, or argon are common choices for these thrusters, though other materials, like magnesium, zinc, and iodine have been experimented with in some designs. The vast majority of ion thrusters rely on gaseous propellants, however.
Electrostatic Thrusters
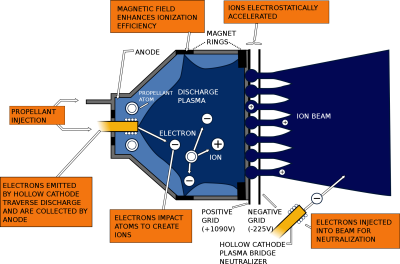
Electrostatic ion thrusters use a variety of methods to accelerate ions to generate thrust. Gridded electrostatic ion thrusters are one of the more popular designs, where the propellant gas is bombarded with electrons to form an ionized plasma. A set of gridded electrodes are then charged with a potential difference, accelerating the positive ions out of the thruster. A separate cathode then discharges low-energy electrons into the exhaust stream of the thruster to ensure the spacecraft doesn’t end up with a net negative charge.
Hall Effect thrusters replace the gridded electrodes with a gas-distributing anode and a magnetically-confined electron cloud acting as the cathode itself. The heavier positive ions are accelerated out of the thruster, while the more lightweight electrons remain confined in the magnetic field. Similarly, a external cathode is used to neutralize the exhaust stream as in the gridded thruster designs.
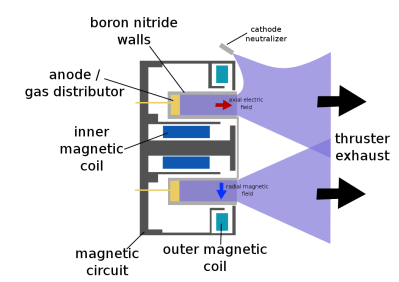
These designs have seen significant use in real-world missions. One of the earliest applications was in Soviet satellites, which used Hall Effect thrusters instead of chemical rockets for station keeping. This is where satellites need to periodically apply thrust over time to counteract the subtle atmospheric drag they experience. The miniscule thrust provided by the Hall Effect thrusters is fine for this purpose, applied over a long period for a significant overall change in velocity. Power draw of these thrusters was on the order of 1.35 kW, generating 83 mN of thrust for a specific impulse of around 1,500-3,000 seconds.
A more recent application of the technology is on the Chinese Tiangong space station, which uses four Hall Effect thrusters to maintain its orbit over time. NASA also hopes to fly the technology on the upcoming Psyche spacecraft, which will use four SPT-140 Hall Effect thrusters. Loaded with 922 kg of xenon propellant, engineers have estimated that 15 times as much propellant would be required if Psyche relied on chemical rockets instead.
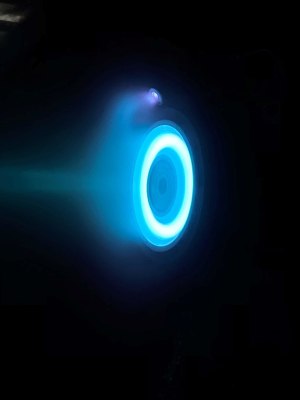
Gridded ion thrusters have seen plenty of use, too. NASA’s NSTAR ion engine was installed on the Deep Space 1 probe, which was sent out to fly by a comet and asteroid in the late 1990s. The gridded ion engine put out just 92 mN of thrust for 2.1 kW of power, but its high specific impulse of 1,000-3,000 seconds enabled significant mass savings compared to a chemical rocket solution for its interplanetary journey. The ion thruster, fueled by xenon gas, ran for a total of 16,265 hours during the mission, providing a total change in velocity (delta-V) of 4.3 kilometers per second, the largest for any spacecraft relying on its own onboard propulsion system.
Other deep space missions have also relied on the technology. JAXA’s Hayabusa probe relied on an ion thruster to help it rendezvous with the Itokawa asteroid. NASA’s Dawn mission also used the technology, being fitted with three of the same xenon ion thrusters used on the Deep Space 1 program, though only firing one at a time in practice. NASA was more than willing to point out the low thrust available from the propulsion system, noting that 0-60 mph would take four days, which compares poorly to the 3.5 seconds achieved by the average modern Ferrari.
Electromagnetic Thrusters
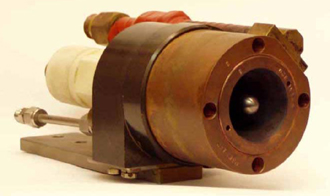
Electromagnetic ion thrusters generate their thrust from neutral plasma, ostensibly consisting of equal numbers of positive ions and negative electrons, and are often referred to as “plasma thrusters” in literature. They come in a variety of designs, most of which use radio energy to ionize gas in a chamber. A magnetic field is then generated to accelerate the overall-neutral plasma out of the thruster. These designs often have the benefit that they don’t need special neutraliziation electrodes to correct the charge imbalance of the exhaust, nor do they use electrodes in the gas stream to accelerate the ions, reducing a source of wear in comparison to electrostatic designs.
One of the most well-developed examples is the VASIMR VX-200 thruster, which has been in development since 2008 in various forms by the Ad Astra Rocket Company. The aim is to operate the thruster at a power level of 100 kW for 100 hours, to indicate how the thruster can generate a huge delta-V for long-term missions. In July 2021, the company reached a milestone of 82.5 kW for 28 hours. The thruster performs with an exhaust velocity on the order of 50 km/s, with a specific impulse of around 5,000 seconds.
Electromagnetic designs often promise larger thrusts than electrostatic thrusters, though most are still in the research stage. Issues with such designs include issues of high power draw and problems of dealing with waste heat. If these could be overcome, designs like a scaled-up VASIMIR electromagnetic thruster could propel a spacecraft from Earth to Mars in just 39 days, compared to the six month journey of a conventional chemical rocket. The only thing is, you’d need a power supply capable of delivering somewhere in the realm of 10 to 20 megawatts of power, and fit that in a spacecraft.
Looking To The Future
Ion thrusters in their various forms are in some ways a technology that haven’t yet proven their full capability. They’ve already done great things, taking small space probes to far-flung destinations while requiring far less fuel along the way. However, we’re still a long way from using them to help us get humans to destinations beyond our own orbit. There’s plenty of development still to happen before you’re riding an ion-powered craft on your future space holiday, but in 50 or a 100 years or so, an ion craft might just be the hot ticket to Mars!
Recommend
About Joyk
Aggregate valuable and interesting links.
Joyk means Joy of geeK