
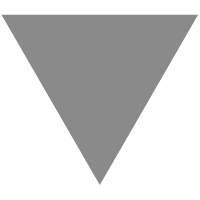
To Invent a Quantum Internet
source link: https://www.tuicool.com/articles/fuqAbui
Go to the source link to view the article. You can view the picture content, updated content and better typesetting reading experience. If the link is broken, please click the button below to view the snapshot at that time.
The first data ever transmitted over Arpanet, the precursor of the internet, blipped from a computer at the University of California, Los Angeles to one at the Stanford Research Institute in Palo Alto on Oct. 29, 1969.
That evening, the team at UCLA got on the phone with the SRI team and began typing “LOGIN.” “We typed the L and we asked, ‘Did you get the L?’” the UCLA computer scientist Leonard Kleinrock recently recalled. “‘Yep’ came the reply from SRI. We typed the O and asked, ‘Did you get the O?’ ‘Yep.’ We typed the G and asked, ‘Did you get the G?’ Crash! The SRI host had crashed. Thus was the first message that launched the revolution we now call the internet.”
The ability of networks to transmit data — as well as their tendency to crash, or otherwise behave unpredictably — has always fascinated Stephanie Wehner . “On a single computer, things will happen nice and sequentially,” said Wehner, a physicist and computer scientist at Delft University of Technology. “On a network, many unexpected things can happen.” This is true in two senses: Programs on connected computers interfere with one another, with surprising effects. And users of networks get creative. With the internet, Wehner noted, initially “people thought we would use it to send around some files.”
Wehner first got online around 1992, a few years before it was easy to do so. A teenager in Germany at the time and already a deft computer programmer, she soon became a hacker on the fledgling internet. At 20, she got a job as a “good” hacker, sussing out network vulnerabilities on behalf of an internet provider. Then she grew bored with hacking and sought a deeper understanding of information transmission and networks.
Wehner is now one of the intellectual leaders of the effort to create a new kind of internet from scratch. She is working to design the “quantum internet,” a network that would transmit — instead of classical bits with values of either 0 or 1—quantum bits in which both possibilities, 0 and 1, coexist. These “qubits” might be made of photons that are in a combination of two different polarizations. The ability to send qubits from one place to another over fiber-optic cables might not transform society as thoroughly as the classical internet, but it would once again revolutionize many aspects of science and culture, from security to computing to astronomy.
Wehner is the coordinator of the Quantum Internet Alliance, a European Union initiative to build a network for transmitting quantum information throughout the continent. In a paper in Science last October, she and two co-authors laid out a six-stage plan for realizing the quantum internet, where each developmental stage will support new algorithms and applications. The first stage is already underway, with the construction of a demonstration quantum network that will connect four cities in the Netherlands — a kind of Arpanet analogue. Tracy Northup, a member of the Quantum Internet Alliance based at the University of Innsbruck, praised “the breadth of Stephanie’s vision, and her commitment to building the kind of large-scale structures that will make it happen.”
After quitting hacking, Wehner went to university in the Netherlands to study computer science and physics. She heard the quantum information theorist John Preskill give a talk in Leiden describing the advantages of quantum bits for communication. A few years later, after earning her doctorate, she left classical bits behind and joined Preskill’s group at the California Institute of Technology as a postdoc.
At Caltech, in addition to proving several notable theorems about quantum information, quantum cryptography and the nature of quantum mechanics itself , Wehner emerged as “a natural leader,” Preskill said, who “was often the glue that bound people together.” In 2014, after a professorship in Singapore, she moved to Delft, where she began collaborating with experimentalists to lay the groundwork for the quantum internet.
Quanta Magazine spoke with Wehner over two days in August. The interview has been condensed and edited for clarity.
The quantum internet is a network for transmitting qubits between distant locations. Why do we need to do that?
The idea is not to replace the internet we have today but really to add new and special functionality. There are all kinds of applications of quantum networks that will be discovered in the future, but we already know quite a number of them. Of course the most famous application is secure communication: the fact that one can use quantum communication to perform what is called quantum key distribution, where the security holds even if the attacker has a quantum computer. A quantum computer would be able to break a lot of the security protocols that exist today.
What makes quantum keys so secure?
A good way to understand what a quantum internet can do is to think about “quantum entanglement,” a special property that two quantum bits can have that makes all of this possible. The first property of entanglement is that it’s “maximally coordinated”: I would have a quantum bit here and you would have a quantum bit in New York, and we would use the quantum internet to entangle these two qubits. And then, if I make a measurement on my qubit here and you make the same measurement in New York, we will always get the same outcome even though the outcome wasn’t determined ahead of time. So you can intuitively think that a quantum internet is very good for tasks that require coordination, due to that first property of quantum entanglement.
Now, given that this is so maximally coordinated, you might say, “Hey, wouldn’t it be great if this entanglement could be shared with hundreds of people?” But that’s actually not possible. So the second property of entanglement is that it’s inherently private. If my qubit here is entangled with your qubit in New York, then we know that nothing else can have any share of that entanglement. And this is the reason why quantum communication is so good for problems that require security.
As one of the simplest applications of quantum communication, quantum key distribution could be available as soon as the early 2020s on the demonstration network you’re building. What are some of the more advanced applications that will become possible later?
New kinds of remote computing will become possible. Say you have a proprietary material design and you want to test its properties in a simulation. A quantum computer promises to be much better at that than a classical computer. But you can imagine that not everybody in the world will have a large quantum computer in their living room anytime soon — possibly not in our lifetime. One way of doing that is you send your material design to me, and I run a simulation for you on my quantum computer and tell you the outcome. That’s great, but now I also know your proprietary material design. So one thing the quantum network makes possible is that you can use a very simple quantum device — in fact, it can make only one qubit at a time — and the quantum network can transfer qubits from your device to my powerful quantum computer. And you can use that quantum computer in such a way that it cannot learn what your material design is while performing the computation.
To give another example, people have also shown that entanglement enables more accurate clock synchronization between two places, which will have a lot of applications. A quantum internet could also be used to make a better telescope, basically by combining distant telescopes. The state of the light particles coming into telescope 1 are teleported, using quantum entanglement, to telescope 2, and then they’re combined with the light of telescope 2.
You’re also working on simulating the future quantum internet. Why is that necessary?
With this very extensive simulation platform we’ve recently built, which is now running on a supercomputer, we can explore different quantum network configurations and gain an understanding of properties which are very difficult to predict analytically. This way we hope to find a scalable design that can enable quantum communication across all of Europe.
The unpredictability of networks is something that has always fascinated me. Computers are interesting, but what I really care about is transmitting data from one point to another. This is the reason why I got into hacking, and why I got interested in the classical internet and gaining access to it in the first place. It’s fundamentally really hard to get a handle on what happens in a network, because there are so many uncharacterized things. For example, if you want to send a message, you cannot predict exactly how long it might take. The message might be lost. A computer might crash. It might go too slow; it might corrupt the data. It might have changed the protocol in unexpected ways because it’s an old version or a new version or a malicious version.
Were you a bad hacker before you became a good hacker?
This is not a thing that one can say in interviews! I think the world was a nicer place back then. But I don’t confess to anything. [Laughs.]
Why did you decide to quit hacking and become a scientist?
I know that hacking sounds super exciting, but I had already done it for some time. Of course one improves methods, but it’s all a little bit more of the same. I got bored and decided to explore some new adventures. And then I discovered quantum information theory and that was super fascinating.
One theorem you went on to prove about quantum information is the noisy storage theorem . What’s that, and what are the implications for quantum communication?
Noisy storage is about cryptography with a physical assumption. In the classical world, one often makes a computational assumption. For example, you assume that it’s difficult to determine the prime factors of large numbers, and if that assumption is true, then my protocol is secure. These security proofs are nice and they’re everywhere, but one should realize that they may be invalidated later. If at any future point someone invents a smart procedure to solve the computational problem that your security is based on, security can be retroactively broken. For instance, when we have quantum computers, they will be able to factor large numbers, and so security based on factoring will be broken. If someone records your messages today, then they may be decrypted later.
The noisy storage work was about: Can we make a physical assumption that can’t be retroactively broken? The physical assumption is that it’s difficult to store a lot of quantum states without noise, which only needs to be true in a very short time frame. If I make the assumption that right now you can only store up to 1 million noisy qubits, then I can treat my protocol parameters to increase security by sending more information than those million noisy qubits can capture. This is nice because if tomorrow you go and buy quantum memory that has 2 million qubits, that’s too late; the information has already been sent securely.
That would allow us to implement all kinds of protocols in quantum communication. Say two people want to compare each other’s passwords without ever giving them away. It’s not like what we do now, when you use an ATM and punch in your PIN there — instead, I’m going to punch in the PIN on my own device, and it will never be leaked to the ATM. That protocol becomes possible with the noisy storage assumption.
Is the pursuit of the quantum internet likely to foster fundamental insights about the laws of nature — a sort of learning-by-doing approach to science?
There’s sometimes a judgment in the sciences that some questions are fundamental and some questions are mundane. I think bringing something into the real world that people can actually use is never mundane. It is extremely hard. There’s this absolutely mind-blowing jump from, “I have this great idea; let’s discuss it on the whiteboard,” to the cellphone that I’m currently using to talk to you. With the quantum internet, we are trying to do this from scratch. From zero. From an early-stage experiment in the lab to this network that we’re trying to set up in the Netherlands, to something that’s outside the lab, that works over distance, that can be used by people, that they can play around with, then by people who don’t need to know physics in order to do it. If one part of the system already existed, we could say, “Now we’re going to improve that.” But the step from zero to the first version is very large.
In doing this, I think we will get a more fundamental understanding in several areas. We will learn more about the physics by making these networks possible because currently we don’t know exactly how to do it. We’re still trying out different kinds of nodes and quantum repeaters, devices that relay entanglement across large distances. And in the domain of computer science, we will learn an entirely new way to program and control such networks due to fundamental differences from classical communication.
But I also think that using such a network, we gain information about creativity and social sciences — about how, in fact, people will go and use these networks. If you look at the classical internet, people thought we would use it to send around some files. That’s great. But people have gotten more creative.
I gather that it’s hard to lay out a timeline for all this, but in your lifetime do you hope to see what you would legitimately describe as a quantum internet?
I would be optimistic about that, yes.
Reprinted with permission from Quanta Magazine , an editorially independent publication of the Simons Foundation whose mission is to enhance public understanding of science by covering research developments and trends in mathematics and the physical and life sciences.
Recommend
About Joyk
Aggregate valuable and interesting links.
Joyk means Joy of geeK